Introduction
The nutritional and functional properties of quinoa natto, a fusion of traditional Japanese natto with quinoa and Thai traditional herbs, were examined. Natto is a traditional Japanese food made by fermenting soybeans with a pure culture of Bacillus subtilis var. natto (Weng et al., 2017). It contains isoflavones, dietary fiber, vitamins, linoleic acid, and various minerals. Additionally, it includes functional compounds such as enzymes, bioactive peptides, nattokinase (a fibrinolytic agent), and gamma-polyglutamic acid (γ-PGA), in a fermentation process that increases nutritional value by enhancing protein digestibility, reducing anti-nutritional factors, and breaking down oligosaccharides like raffinose and stachyose (Sanjukta & Rai, 2016; Weng & Chen, 2010, 2011). Quinoa (Chenopodium quinoa Willd.) is a tetraploid herbaceous plant that is grown as a halophytic crop. It belongs to the Dicotyledoneae class, Chenopodiaceae family, and Chenopodium genus. Quinoa is a unique food that supports human nutrition with its rich amino acid, iron, calcium, magnesium, and fiber content (Jiang et al., 2024; Sezgin & Sanlier, 2019; Tanwar et al., 2019; Vidueiros et al., 2015). Many herbs possess antioxidant properties for protection against disease, such as garlic, turmeric, and curry powder. Garlic (Allium sativum L.) is a cultivated plant that can help treat the ailments of many people (Alam et al., 2016) and increase immunity. It has useful medicinal properties, including anti-inflammatory, anti-cancer, anti-obesity, antifungal, immunomodulatory, and renal protective activities in both raw and cooked forms (Shang et al., 2019; Stępień et al., 2024; Zhang et al., 2015) as well as in fermented garlic (Paulauskienė et al., 2024; Tahir et al., 2022). There are many bioactive compounds in turmeric including polyphenols, flavonoids, tannins, and ascorbic acid (Gopinath & Karthikeyan, 2018; Tanvir et al., 2017). Curcumin, a substance in turmeric, has antioxidant, anti-inflammatory, and anti-apoptotic properties (Barnwal et al., 2014; Basuny et al., 2023; Permatananda et al., 2021; Rahbardar & Hosseinzadeh, 2024). Curry powders are used as spices in Asian countries such as Pakistan, India, Thailand, Taiwan, Sri Lanka, and Japan (Tayyem et al., 2006). They contain antioxidants derived from various spice ingredients. Curcumin is contained in turmeric. It is a powerful antioxidant known for its anti-inflammatory properties. Turmeric (Curcuma longa) of Bangladesh has antioxidant properties that arise from its polyphenol, flavonoid, tannin, and ascorbic acid contents (Jo & Surh, 2020; Sandur et al., 2007; Tanvir et al., 2017). Spice combinations in curry powder provide a source of diverse antioxidants, each contributing to health benefits (Jo & Surh, 2020; Petchlert et al., 2014; Sandur et al., 2007; Siripongvutikorn et al., 2024). The phenols and flavonoids in curry powder come from various spices, such as turmeric, cumin, coriander, black pepper, and ginger.
Consequently, the current study aims to develop quinoa natto products supplemented with herbs (turmeric, garlic, and curry). The resulting products are evaluated for their physicochemical characteristics and protein changes in terms of total soluble proteins (TSP), trichloroacetic acid (TCA)-soluble peptides, and amino acid profiles. Furthermore, this study evaluates and predicts the biological properties of NQP peptides and essential amino acids through in silico antioxidant and antimicrobial activities. This is done to model antimutagenic and antimicrobial agents using a SwissDock-molecular docking server.
Materials and Methods
Raw materials and starter culture
In this research, we used quinoa (Chenopodium quinoa Willd.) as the main ingredient instead of soybeans, purchased from a local market in Ubon Ratchathani, Thailand. A commercial natto product (Natto Tsubaki Mini, Tsubaki Food Service, Japan) was used as a starter culture of Bacillus subtilis subsp. natto NARUSE.
Fermentation process of quinoa natto (QN)
Before the fermentation, quinoa was cleaned with distilled water and steamed by autoclave sterilization at 121 °C for 15 min. After cooling, the fermentation process was started by mixing 20% (w/w) of an industrial natto product, a cultured B. subtilis subsp. natto (at an initial cell count of ≤ 107 CFU/mL), into steamed quinoa. Next, 500 g of the mixture was packed in a zip-lock plastic bag and incubated at 37 °C, followed by collecting three replicate samples at 0, 24, and 48 h after the start of fermentation, which is a slightly modified procedure from Punyauppa-Path et al. (2024).
Preparation of quinoa natto supplemented with herbs (QNHs)
This study used Thai herbs, specifically turmeric, garlic, and curry powders obtained from a local market in Ubon Ratchathani, Thailand. They were used to improve quinoa natto's food quality and boost its nutritional values in terms of its antioxidant content. After 48 h of fermentation, the quinoa natto proteins (QNPs) were used to formulate QNHs by supplementation with 0.02% (w/w) of different herbs. The groups are (F1): QNP+ turmeric powder, (F2): QNP+ garlic powder, (F3): QNP+ curry powder, and (F4): QNP+ mixed herb powder (Figure 1). These samples were mixed in a blender at 6,500 rpm until a homogenous mixture was attained. After that, the mixture (approximately 350 g) of QNHs was placed in a covered plastic container and stored at 4 °C until further use.
Figure 1
The appearance of quinoa natto samples during the fermentation process and development of their products. They are formulated to produce quinoa natto proteins (QNPs) supplemented with Thai herbs. Specifically, F1 represents QNP+ turmeric powder, F2 is QNP + garlic powder, F3 represents QNP + curry powder, and F4 is QNP + mixed herb powder.
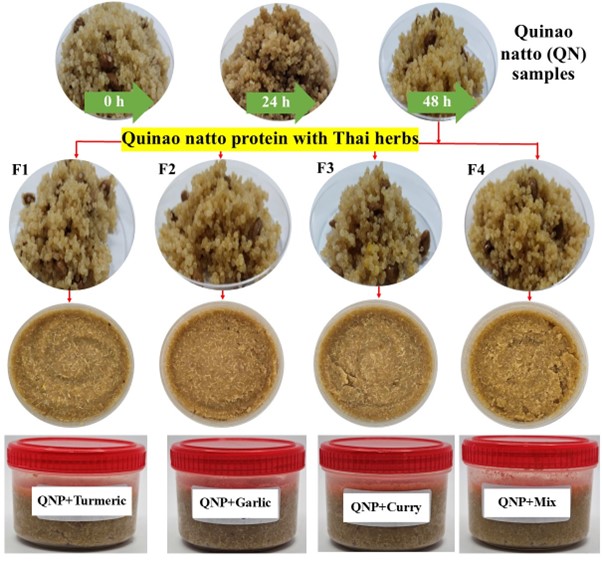
Protein extraction of QN and QNH products
Extraction of the QN and QNH products was conducted using microwave-assisted extraction to prepare QNPs and QNPH samples, following a modified method outlined by Phupaboon et al. (2023) and Punyauppa-Path et al. (2024). In summary, a product weighing 10 g was placed into 100 mL of PBS buffer (pH 6.4) and was subjected to homogenization and extraction using microwave equipment. The process was done under optimal conditions, at a 100 W power and a maximum temperature of 60 ºC for 10 min. Subsequently, a homogenate from each sample underwent centrifugation at 10,000 rpm and 4 ºC for 5 min. The resulting supernatant was then filtered to produce QNPs and QNPH extracts. The extracted supernatants were kept at a constant temperature of 4 °C while their microbiological, physicochemical properties and bioactivities were examined.
Characterization of QNPs and their QNPHs
pH analysis and microbial counts
Filtered supernatant was directly analyzed using a pH meter according to AOAC (2000). Total bacterial counts were done using a pour plate technique and data was recorded as log CFU/mL according to the procedure of Phupaboon et al. (2022a).
Total soluble protein (TSP) and trichloroacetic acid (TCA)-soluble peptide analyses
In the TSP and TCA-soluble peptide analysis, 1 mg of QNPs and QNPHs were uniformly dissolved in 1 mL of distilled water and a 10% (w/v) TCA solution, following a process described by Phupaboon et al. (2022c). The pertinent values were obtained utilizing the Lowry method (Lowry, 1951) and the total soluble protein (TSP) content was represented as mg BSA/g of dry extract (DE), measured at a 660 nm wavelength. TCA-soluble peptides were quantified based on the glycine content as a mg/g DE, measured spectroscopically at 595 nm with the Folin-Ciocalteu reagent. These measurements were obtained from triplicate experiments.
Sodium dodecyl sulfate-polyacrylamide gel electrophoresis (SDS-PAGE) analysis
SDS-PAGE was used with a Mini-Protein II-unit (Bio-Rad, CA, USA) to examine the changes in QNPs and QNPH extracts, as discussed by Phupaboon et al. (2020). SDSPAGE used an acrylamide gel with stacking (5%) and resolving (12%) gels, produced using distilled water, 1.5 M Tris HCl at pH 8.8, 10% SDS, 30% acrylamide-bis, 10% ammonium persulfate, and TEMED, followed by a protein marker (Chromatein Prestained Protein Ladder, Vivantis, Malaysia). All chemical reagents were of molecular biology quality and purchased from Merck, USA.
Total polyphenol, total flavonoid, and antioxidant analyses
Determination of the Total Polyphenolic Content (TPC), Total Flavonoid Content (TFC), and antioxidant capacity in terms of DPPH, ABTS, and FRAP methods of the QNPs and QNPH extracts was done using a 96-well microplate assay employing an EnSight multimode plate reader (PerkinElmer Inc., Waltham, MA, USA). The protocol described by (Phupaboon et al., 2022b) was followed for TPC determination (as mg GAE/g DE) using the Folin–Ciocalteu reagent with absorbance at 765 nm and TFC evaluation (as mg QUE/g DE) using a 10% aluminum chloride solution read at 415 nm. Additionally, assessment of antioxidant activity (as mg TROE/g DE) was conducted using three distinct methodologies, the DPPH reagent read at 517 nm, ABTS reagent read at 734 nm, and the completed FRAP reagent read at 593 nm. All analyses were conducted in triplicate.
Amino acid analysis
Amino acid contents were analyzed using thin-layer chromatography (TLC) densitometry, with a slightly modified methodology from the protocol of Rajurkar and Gaikwad (2014). In sample preparation, the obtained QNPs and QNPHs were weighed and dissolved in PBS buffer (pH 6.5) to obtain a concentration of 30 mg/mL. Additionally, the amino acid standard for TLC analysis at a 98% purity (Sigma-Aldrich, USA) consisted of L-lysine, L-leucine, L-valine, and L-threonine. It was prepared using two-fold dilution in methanol to obtain initial concentrations of 62.5–1000 µg/mL. In analysis, 20 µL of each sample and amino acids were spotted onto TLC plate silica gel 60 F 254 (Merck, Singapore) using an automatic TLC spot (CAMAG Linomat IV, Muttenz, Switzerland) with a distance from the base of 1.5 cm and 1 cm from the side. The bandwidth was set to 8 mm. After running the TLC plate to the specified solvent front (8 cm), the mobile phase used n-butanol: acetic acid: water in a ratio of 6:1.5:2.5. Subsequently, the TLC plate was dried in an oven at 65 °C for 10 min and sprayed with the ninhydrin reagent. Next, the plate was analyzed for colored spots using a CAMAG TLC scanner III at a 495 nm wavelength under a D2 lamp and linked with the densitogram from the winCATS Planar Chromatography Manager program to determine the area under the curve (AU).
Molecular docking study
This investigation is predicated on the potent antioxidant and antibacterial efficacy of essential amino acids such as L-leucine, wherein L-leucine serves as the principal biological agent. L-leucine compounds (PubChem ID: 6106 and SMILES ID: CC(C)C[C@@H](C(=O)O)N) were chosen as ligand molecules for initial screening and to forecast the binding site of the energy-minimized target protein structure utilizing the SwissDock server within the SwissDrugDesign platform, adhering to the methodologies and parameter configurations outlined by Bugnon et al. (2024). For ligands generated using the updated version of SwissParam utilizing a simplified molecular input line entry system (SMILES), notations were sourced from the PubChem database. Subsequently, among the selected four active site protein targets— type-1 angiotensin II receptor (PDB ID: 6OS1), parathyroid hormone-related protein (PDB ID: 3FFD), 3-oxoacyl-[acyl-carrier-protein] synthase1 from E. coli (PDB ID: 1FJ4), and nucleoside diphosphate kinase from S. aureus (PDB ID: 3Q8U)—three-dimensional (3D) structures were elucidated using SWISS-MODEL and retrieved from the RCBS-PDB database. The docking of L-leucin with protein targets was performed using the SwissDock version 2024 free server (http://www.swissdock.ch/) (accessed on 28 October 2024), employing the EADock DSS algorithm under Attractive Cavities 2 (AC 2). The AC 2 in SwissDock version 2024 employs the Vina Python library (version 1.2.5) to create a Vina sampling engine and utilize the scoring functions of AutoDock4, which encompasses the AC docking score (comprising the CHARMM force field energy and FACTS solvation energy components) and the SwissParam score (an estimation of the binding free energy).
Statistical Analysis
Each evaluation in this study was conducted three times. The data is displayed as the mean value plus or minus the standard deviation. Analysis of Variance (ANOVA) was done using SPSS-KKU Statistics v.27 to evaluate the disparities across the treatments. Duncan's multiple range tests (DMRTs) were employed, with a significance level set at p<0.05.
Results
Physicochemical and microbiological characteristics of QNPs and QNPHs
Figure 2
pH changes and total microbial counts during QN fermentation and their sample formulation. Error bars indicate the SD of the mean (n=3).
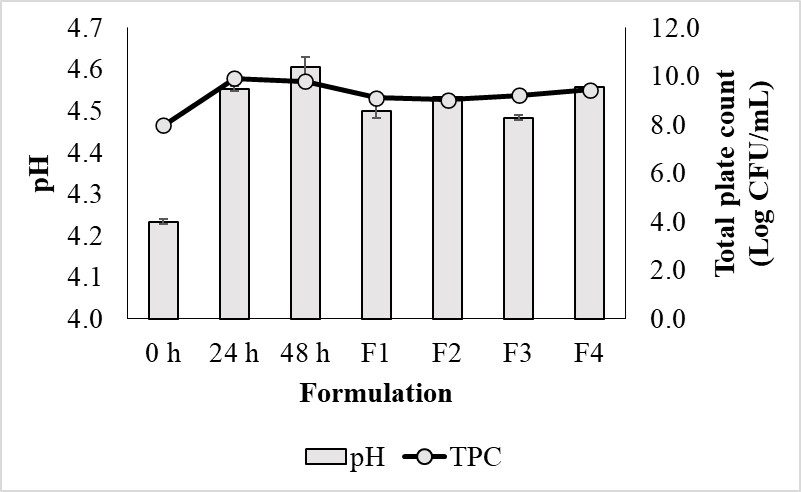
The highest level of TPC in quinoa natto was recorded on day 2 of fermentation. At 48 h, the bacteria grew best with a value of 10 log CFU/mL in both quinoa natto and F4. However, the pH increased in the first 24 h. The pH changed little (Figure 2).
Protein alteration of QNPs and QNPHs
Figure 3
Protein alteration of QNPs and QNPHs determined by physicochemical characteristics (a) and analyzed protein profiles using SDS-PAGE with different formulations. Error bars indicate SD of the mean (n=3), while * indicates significant differences (p < 0.05) between the mean value of each formulation.
Changes in TSP and TCA are illustrated in Figure 3a. The initial TSP was 230 mg/g. TSP changes during quinoa natto fermentation were determined using SDS-PAGE. The total soluble protein of the quinoa natto fermentation was measured at various times from day 0 to 24. Total soluble protein decreased with fermentation time. The lowest expression of total soluble protein occurred on day 2 of fermentation (Figure 3a). TSP values of F1, F2, and F3 are similar, but the TSP value of F4 is the lowest.
After natto was incubated and fermented for various times, the total protein was extracted and determined using SDS-PAGE. For natto fermented with or without the herb ingredient mixture, free amino acids/peptides with a 5 kDa of molecular weight were detected. The natto fermented without the herb ingredient mixture and incubated for 48 h showed colored band sizes that appeared in the experiment from 0 to 24 h.
The natto in group F1 presented less free amino acids/peptides than the F2, F3, and F4 groups when it was fermented with or with no herb ingredient mixture. Natto in group F4 exhibited bands with greater protein intensity than the natto fermented without a herb ingredient mixture (Figures 3a, 3b). After determining the structural subunit of natto protein characterization, the β-basic subunit was presented at 10 kDa in all natto groups whose bands at 0 h were more intense than at 24 and 48 h of incubation. At 48 h of incubation, natto fermented without the herb ingredient mixture showed a band intensity that was not different than with the herb ingredient mixture. The α-acidic subunit of natto fermented without the herb ingredient mixture was detected at 62 kDa after a 48 h incubation. This band was more intense than at 0 to 24 h. Band intensities of F1, F2, and F3 were not different from samples with no herb ingredient mixture. However, the F4 group showed less band intensity than natto fermented for 48 h with no herb ingredient mixture. After natto fermentation for various times, the globulin structural subunit might degrade into small molecules of free amino acids/peptides in a time-dependent manner. The F4 group demonstrated a greater amount of free amino acids/peptides than with the herb ingredient mixture (Figure 3a). In the F4 group, the total soluble peptide value was 600 mg/g, which is significantly more than natto fermented with or without the herb ingredient mixture in groups F1, F2, and F3. However, as fermentation time was increased from 0 to 24 h, the amount of total soluble peptides decreased. After blending herbs into the natto with the same fermentation time, the natto in group F4 exhibited a higher amount of total soluble peptides than groups F1, F2, and F3 (Figure 3a).
Bioactive compounds and antioxidant activities of QNPs and QNPHs
Table 1
Biological activity of QNPs and QNPHs at different fermentation stages and formulations.
Values are expressed as the mean ± SD (n=3) in each column, which indicates different levels of biological activity, and different a-d superscripts indicate a significance level at p<0.05 by Duncan’s test.
The highest amount of TPC was 102.6±0.8 mg GAE/g after 48 h of fermentation in F1, while the lowest was 75.0±1.4 mg GAE/g in F3. The total flavonoids were analyzed and quantified as was the total phenolic content (TPC). The lowest quantity of total flavonoids was 4.7±0.3d TFC (mg QUE/g), while the highest amount was 8.2±0.0 after 48 h of fermentation in F3. Additionally, the free radical scavenging efficacy of fermented quinoa natto was demonstrated through DPPH and ABTS assays.
The total phenolic compounds (TPC) and total flavonoids (TFC) of natto fermented with or without herb ingredients as a function of time and type of herbs were measured. TPC and TFC increased with fermentation time. At 48 h of fermentation, natto presented higher amounts of both TPC and TFC than at 0 and 24 h. Comparing groups fermented with and without the herb ingredient mixture, the F3 natto presented significantly higher TPC and TFC levels than other groups (Table 1). DPPH and ABTS methods were used to investigate the free radical scavenging activity. At 24 and 48 h of fermentation, natto showed higher activity than the unfermented group. After 48 h, the natto fermented group showed 77.2±1.3% free radical scavenging, while at 0 h, this activity was 63.1±1.2%. When comparing groups fermented with and without the herb ingredient mixture, the F4 natto exhibited significantly more scavenging activity than the F1, F2, and F3 groups, 85.3±6.0%. The F3 group showed 72.0±1.8% scavenging activity, which is higher than in the F1 and F2 groups, 66.1±2.7% and 68.4±4.3%, respectively. Using the ABTS method, natto fermentation time affected the free radical scavenging activity (Table 1). At longer natto fermentation times, more scavenging was observed. After 24 and 48 h of fermentation, higher activity was observed than at 0 h. When comparing fermented samples with and without the herb ingredient mixture, all natto samples fermented with herbs had more free radical scavenging activity, F1 (59.1±1.8%), F2 (58.0±1.5%), F3 (58.0±1.6%), and F4 (55.7±0.6%). For inhibition of the FRAP antioxidation reaction, natto fermentation time did not affect free radical scavenging activity. The F3 sample presented the highest FRAP antioxidative activity, 22.5±0.8% compared to F2 (17.6±1.2%) and (12.7±0.6%), respectively. For assessment of total free radical scavenging potential, the F3 group natto demonstrated the highest potentials as greater amounts of TPC and TFC represent activity against the free radicals.
Amino acid profiles of QNPs and QNPHs
Table 2
Concentrations of amino acids obtained from QNPs at 48 h after incubation and different QNPH products.
The values in Table 2 are expressed as the mean ± SD (n=3) in each column, which show the amino acid contents, and the a-d superscripts indicate a p<0.05 significance level by Duncan’s test. The study on the amino acid concentrations of QNPs at 48 h and QNPH blended with different herbs using the TLC method showed that QNPH F3 had a significant amount of L-lysine (99.2±27.2) µg/spot, which was significantly higher than that of QNPH F4, F2, F1, and QNPH at 48 h (p<0.05). The L-valine content in QNPH F3 was (16.0±50.6) µg/spot, which was significantly higher than QNPH F4, F1, QNPs at 48 h, and QNPH F1 (p<0.05). QNPH F3 also showed an L-leucine content of 356.4±516.0 µg/spot, significantly higher than QNPs at 48 h, QNPH F1, F4, and F2 (p<0.05). QNPH F3 exhibited an L-threonine content of (80.0±264.9) µg/spot, which was significantly higher than QNPH F4, F2, and F1. These results are summarized in Table 2.
It can be concluded that natto QNPH F3 contained significantly higher amounts of L-valine, L-threonine, L-lysine, and L-leucine, 16.0±50.6, 80.0±264.9, 99.2±27.2, and 356.4±516.0 µg/spot, respectively, compared to QNPH F4, F2, and F1, (p<0.05). The concentrations of these amino acids were analyzed in terms of mg/g of dry extract.
QNPH F3 had an L-lysine content of 122.8±6.0 mg/g dry extract, which was significantly higher than QNPH F4, F2, F1, and QNPs at 48 h (p<0.05). The L-valine content of QNPH F3 was 26.7±5.2 mg/g dry extract, higher than QNPH F4, F1, QNPs at 48 h, and F2. QNPH F3 had an L-leucine content of 594.0±39.4 mg/g dry extract, higher than F4, F2, F1, and QNPs at 48h. The L-threonine content was 133.4±26.2 mg/g dry extract in QNPH F3, significantly higher than that of QNPH F4, QNPs at 48 h, F2, and F1 (p<0.05). The levels of L-lysine, L-valine, L-leucine, and L-threonine in mg/g dry extract in natto showed that QNPH F3 respectively contained 26.0±5.2, 122.8±6.0, 133.4±26.2, and 594.0±39.4 mg/g dry extract. These values are statistically higher than those of QNPH F4, F2, F1, and QNPs (p<0.05), as shown in Table 2.
The current study concluded that among both plain natto quinoa and natto quinoa blended with various herbs and fermented for 48 h, natto QNPH F3, which was mixed with curry, had higher concentrations of L-valine, L-lysine, L-threonine, and L-leucine in terms of mg/g dry extract than natto quinoa mixed with herbs such as turmeric, garlic, mixed herbs, and plain natto quinoa.
Molecular docking profile
Table 3
Molecular docking analysis results and 3D docking structure of L-leucine ligand in various active sites (protein PDB) of type-1 angiotensin II receptor (a); parathyroid hormone-related protein (Caco-2 cell protein receptor) (b); 3-oxoacyl-[acyl-carrier-protein] synthase1 of E. coli (c); and nucleoside diphosphate kinase of S. aureus (d).
Figure 4a
3D docking structure of L-leucine ligand in various active sites (protein PDB) of type-1 angiotensin II receptor
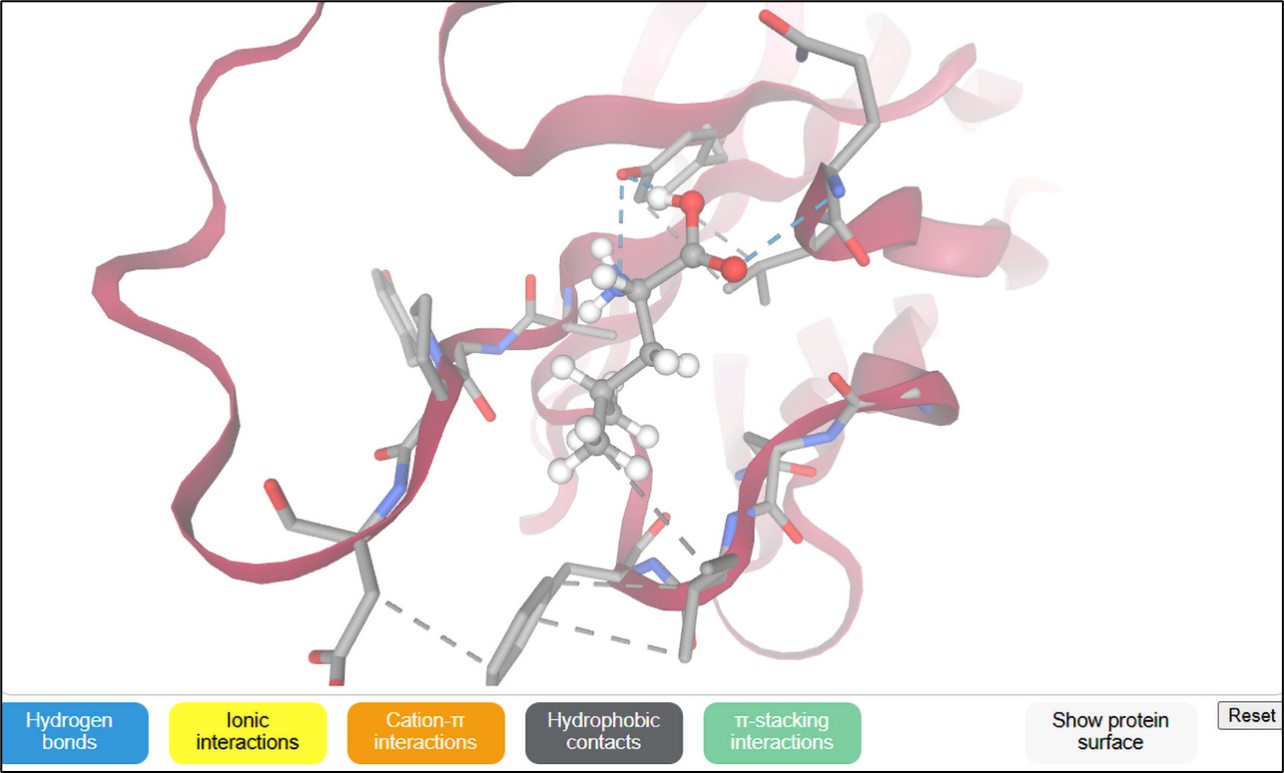
Figure 4b
3D docking structure of L-leucine ligand in various active sites (protein PDB) of parathyroid hormone-related protein (Caco-2 cell protein receptor)
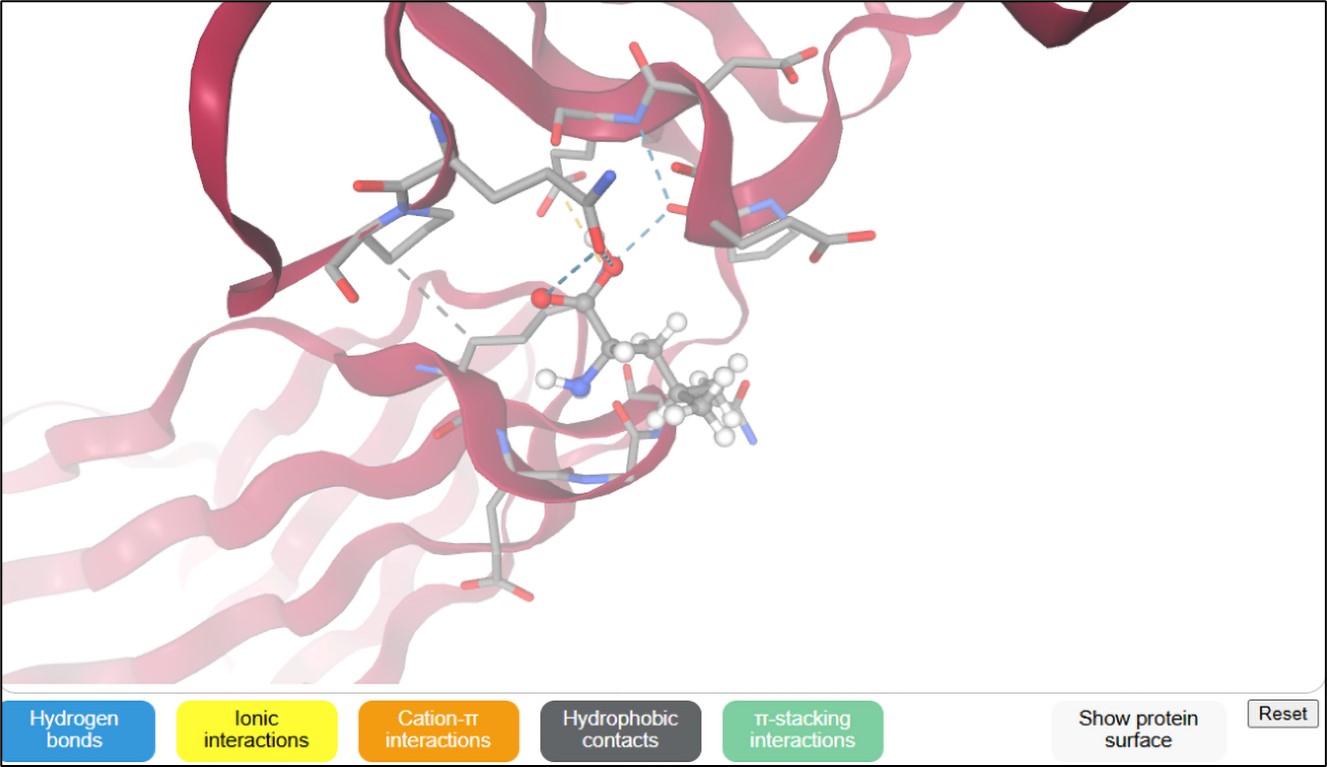
The binding interactions of L-leucine as a ligand molecule [SMILES ID: CC(C)C[C@@H](C(=O)O)N] were docked at several protein active sites using the SwissDock server, as presented in Table 3 and Figure 4(a-d). These interactions involve hydrogen bonds, ionic bonds, cation contacts, hydrophobic interactions, and stacking interactions. The AC docking scores of the L-leucine ligand vary from -6.10 to -9.15 kcal/moL docked four PDB-protein targets (6OS1, 3FFD, 1FJ4, and 3Q8U) of mutagenic receptor and protein synthesis-pathogenic bacteria. Specifically, in 3FFD, the greatest AC docking score of -9.15 kcal/moL was seen against the parathyroid hormone-related protein from the Caco-2 cell protein receptor, followed by -7.65 kcal/moL with the type-1 angiotensin II receptor from hypertension. The SwissParam score, representing the binding free energy derived from the weighted sum of polar and nonpolar contributions, exhibited high docking scores of -5.59 kcal/moL interacting with the type-1 angiotensin II receptor. Other protein targets yielded scores of -5.52, -5.45, and -5.32 kcal/moL, corresponding to 3-oxoacyl-[acyl-carrier-protein] synthase1 of E. coli, nucleoside diphosphate kinase of S. aureus, and the parathyroid hormone-related protein from the Caco-2 cell protein receptor, respectively.
Discussion
The highest total phenolic content (TPC) was 102.6±0.8 mg GAE/g after 48 h of fermentation, observed in QNPs with turmeric powder. The results of this research are consistent with Güneri (2021). The lowest TPC was 75.0±1.4 mg GAE/g in QNPs with curry powder. Total flavonoids were also measured, with the lowest level (4.7±0.3 mg QUE/g) in unfermented quinoa natto, and the highest (8.2±0.0 mg QUE/g) in QNPs with curry powder after 48 h of fermentation. These results are consistent with Petchlert et al. (2014), Jo and Surh (2020), Siripongvutikorn et al. (2024) and Sandur et al. (2007). In the research, it was found that the quantity of QNPs with curry powder after 48 hours of fermentation was the highest. The researchers observed that curry powder contains a variety of ingredients, especially turmeric, which led to a positive response in antioxidant presence. This finding aligns with previous research showing that turmeric and curcumin are associated with antioxidant properties (Jo & Surh, 2020; Sandur et al., 2007; Tanvir et al., 2017). The researchers also noted that turmeric alone showed high antioxidant effects, likely due to its natural antioxidant compounds. However, the antioxidant levels were somewhat lower, possibly because turmeric is a single ingredient, while curry powder consists of multiple components that also contain antioxidants, such as chili and ginger.
The current study also evaluated the antioxidant properties of fermented quinoa natto using DPPH and ABTS assays. Additionally, the TPC and total flavonoid content (TFC) in natto increased with fermentation time. After 48 h, the levels of both TPC and TFC were higher than at 0 and 24 h. Among the groups of QNPs with curry powder, those fermented with a mixture of herb ingredients showed significantly higher TPC and TFC values. Fermented natto at 24 and 48 h exhibited higher free radical scavenging activities than the unfermented group. After 48 h, the free radical scavenging activity reached 77.2±1.3%, compared to 63.1±1.2% for the unfermented group. The ABTS method confirmed that longer fermentation enhanced free radical scavenging activity, with all herb-fermented natto groups showing higher activity than those with no herbs. In terms of antioxidative response measured by the FRAP method, fermentation time did not significantly impact activity. Natto quinoa mixed with curry powder showed the highest antioxidative reaction (22.5±0.8%), compared to QNPs with garlic powder (17.6±1.2%) and QNPs with turmeric powder (12.7±0.6%). Overall, QNPs with curry powder exhibited the greatest potential for free radical scavenging, indicated by higher TPC and TFC levels, reflecting its effectiveness against free radical production.
The findings of the current study are consistent with those of Saito et al. (2003) and Kim et al. (2020) who reported free amino acids directly contribute to several health benefits, including the reduction of blood cholesterol, antimutagenicity, decreased risk of coronary heart disease, and antiobesity potential. Furthermore, certain amino acids, including Tyr, Met, His, Lys, and Trp, are recognized for their antioxidant properties, whereas soy glycopeptides, including Asp, Glu, Pro, Gly, and Leu, exhibited significant cytotoxic effects on cancer cells and antimicrobial activity. Currently, molecular docking approaches are vital tools for identifying effective compounds in the virtual screening of large databases, focused on predicting protein-ligand interactions related to antimutagenic and antimicrobial activities (Snoussi et al., 2022). Kharpan et al. (2024) successfully synthesized and characterized new copper(II) and zinc(II) complexes derived from smectogenic L-leucine methyl ester Schiff base ligand, elucidating their binding interactions with biomolecules such as CT-DNA and BSA. The compounds were evaluated for their in-vitro anti-parasitic efficacy against Leishmania donovani utilizing the MTT assay. All the compounds demonstrated IC50 values ranging from 31.29 to 57.01 μM. Molecular docking was conducted to elucidate the binding characteristics of the compounds with CT-DNA. Additionally, Sharifi et al. (2013) conducted an investigation into the structure of a pure compound, which was isolated from O. acanthium and demonstrated an ACE inhibition activity of 83±1% at a concentration of 330 μg/mL. The isolated compound exhibited commendable antioxidant activity, with an IC50 value of 2.6±0.04 μg/mL, when juxtaposed with BHT, which demonstrated an IC50 value of 10.3±0.15 μg/mL, and Trolox, presenting an IC50 value of 3.2±0.06 μg/mL. The predictions from molecular docking indicated a competitive type of enzyme inhibition, revealing an approximately comparable affinity of the isolated compound for both the ACE-C and N domains.
Conclusions
This study described the physicochemical characteristics of total phenolic content (TPC) and total flavonoids (TFC) in QNPs, which increased with optimal fermentation conditions (48 h). The biochemical properties showed that the highest TPC was in QNPs with turmeric powder (F2), while the lowest was in QNPs with curry powder (F3). The total phenolic compounds (TPC) and total flavonoids (TFC) of natto fermented with or without herb ingredients as a function of time and type of herbs were measured. TPC and TFC increased with fermentation time. At 48 h of fermentation, natto presented higher amounts of both TPC and TFC than at 0 and 24 h. Comparing groups fermented with and without the herb ingredient mixture, the F3 natto presented significantly higher TPC and TFC levels than other groups. The investigation revealed that in silico docking of L-leucine ligands for antioxidant capacity and antimicrobial potential could play a crucial role in functional food as a therapy to overcome bacterial resistance and antimutagenic cells. Therefore, this product would provide supportive information for further investigation via in vivo study and their evaluation of sensory tests.
Acknowledgements
No particular funding from public, commercial, or nonprofit organizations was obtained for this research.
Author Contributions
SPu conceived and designed the study. SPh, PK, PT, SPi and UT carried out data collection, integrated the inventory, and perfomrmed the analysis, as well as contributed to manuscript writing. SPu, JS, NS, PP, and PR reviewed and edited the manuscript. All authors have read and approved the final published version.