INTRODUCTION
Type II Diabetes, a metabolic condition recognized by increased blood glucose levels after meals, is expected to undergo a likely surge in affected individuals. Optimal management of blood glucose levels is crucial, not just to prevent the progression of T2DM but also to alleviate micro- and macrovascular complications (Gandhi & Mooradian, 2022). Slowing down the absorption of glucose after meals, an approach aimed at reducing post-meal hyperglycemia, involves inhibiting α-glucosidase. This delay in glucose absorption is recognized as advantageous in disease treatment. Medications like acarbose and voglibose, known as α-glucosidase inhibitors used in diabetes treatment, come with side effects like flatulence and diarrhea (Dirir, Daou, Yousef, & Yousef, 2022). Hence, the quest for alternative drugs, potentially from new sources like Thai herbs, is underway to minimize these side effects. Research has identified that individuals with diabetes are more susceptible to infections within their bodies compared to the general population (Agrawal, Sharma, Singh, & Goyal, 2022; Khwaja & Arunagirinathan, 2021). Previous studies highlight a high likelihood of infectious diseases among those with diabetes, particularly concerning the flu and bloodstream infections. These studies indicate that individuals with diabetes are three to five times more likely to contract influenza than the general population, and they also face an increased risk of recurrent bacterial infections (Anhê et al., 2020; Daryabor, Atashzar, Kabelitz, Meri, & Kalantar, 2020; Wensveen, Gašparini, Rahelić, & Wensveen, 2021).
Currently, there is growing interest in utilizing plant extracts for the treatment of diabetes. Numerous plants are abundant in various natural antioxidants, including tannins, flavonoids, as well as vitamins C and E. These compounds have the ability to support the function of β-cells and lower glucose levels in the bloodstream. However, the extraction process from plants is crucial as it determines the yield and specific bioactive substances obtained. Traditional methods, such as maceration and Soxhlet extraction, involve lengthy procedures and significant solvent usage, raising concerns about energy consumption and the extraction's overall value. Microwave-assisted extraction (MAE) is a popular and cost-effective method for extracting bioactive compounds from medicinal plants. It utilizes microwaves and organic solvents to heat from the inside out, enhancing extraction efficiency. MAE has been proven reliable, stable, and capable of continuous extraction. Studies show its effectiveness in extracting phenolics, flavonoids, terpenoids, saponins, and essential oils, providing higher yields compared to traditional methods (Bagade & Patil, 2021; Lasunon & Sengkhamparn, 2022; Silva, Dias, & S, 2022).
Pepper (Piper nigrum Linn.) of the Piperaceae family is a widely recognized spice used in various global cuisines, boasting an array of bioactive compounds with numerous advantageous medical properties. The primary pharmacological element in pepper is piperine, an alkaloid known for its diverse effects, including antimicrobial, anticancer, and antiglycan properties (Haq et al., 2021). Piperine is responsible for the distinctive aroma of black pepper and contributes to its antioxidant attributes. Research indicates that treatment with piperine or black pepper can suppress reactive oxygen species, boost antioxidant molecules, and reduce cellular thiol status, antioxidant enzymes, and lipid peroxidation (Stojanović-Radić et al., 2019). Additionally, piperine enhances the absorption and bioavailability of intestinal brush border and phytochemicals, thereby augmenting the medicinal benefits of drugs. The combined use of black pepper is likely to enhance the antidiabetic effects of other spices, as piperine intensifies the phytochemical benefits (Chopra, Saini, & Dhingra, 2023). Moreover, piperine, when present at a concentration of 4-6%, effectively stimulates peroxisome proliferators-activated receptor-gamma (PPAR-g), acting as an insulin hormone receptor on the surface of muscle cells and liver cells, akin to thiazolidinediones (TZDs), a drug group (Yan et al., 2019). Studies also suggest that piperine inhibits α-glucosidase from baker's yeast, with an IC50 value of 0.9 µg/mL, potentially reducing post-prandial glucose levels (Haguet et al., 2023). (Duan, Xie, Yu, Wang, & Yang, 2022) propose that the immunomodulatory effect of pepper may arise from a combination of humoral and cell-mediated immune responses. Notably, research has predominantly focused on generic pepper varieties. Grown in Trang province, southern Thailand, Trang pepper has received a Geographical Indication (GI) due to its unique olfactory profile, characterized by a refreshing aroma, distinct flavor, and robust spiciness. This heightened spiciness is attributed to notably higher concentrations of piperine (5-9%) compared to other pepper cultivars. Traditional medicine has found that Trang peppercorn helps reduce tension, fat, and blood sugar levels. It can also boost the immune system (Liamnimitr, Hansuek, & Unjan, 2023). Currently, there is a lack of specific investigations regarding Trang pepper's effectiveness in reducing blood sugar levels or its potential to enhance the immune system. Therefore, this study was conducted to explore the immune-stimulating and α-glucosidase inhibitory activities of three types, namely black (BP), red (RP), and white (WP) pepper of Trang pepper using MAE technique. The findings of this research are anticipated to contribute to the development of bioactive-rich herbal medicine for diabetes therapy and immune stimulation.
MATERIAL AND METHODS
Chemicals and reagents
Acetic acid, aluminum chloride, acetonitrile, dimethyl sulfloxide (DMSO), Dulbecco’s modified eagle’s medium (DMEM), Folin-Ciocalteu reagent, hydrochloric acid, methanol, maltose, potassium acetate, potassium hydroxide, phosphate buffer, sodium carbonate, sodium hydroxide, sodium chloride, and sucrose were purchased from Merck (Darmstadt, Germany). Baker’s yeast, fetal bovine serum, gallic acid, lipopolysaccharide (LPS), nitroblue tereazolium (NBT), nitric oxide assay kit, p-nitrophenyl-a-D-glucopyranoside (pNPG), piperine, penicillin/streptomycin, quercetin, resazurin, rat intestinal acetone powder, and zymosan A from Saccharomyces cerevisiae were purchased from Sigma-Aldrich (St. Louis, MO, USA). The glucose kit was purchased from Human (Magdeburg, Germany). Acarbose (Glucobay®, 100 mg) was purchased from Bayer Healthcare (Leverkusen, Germany).
Trang peppercorn preparation
The red shoot strain of Trang pepper used in the study was obtained by collecting BP, RP, and WP peppercorn in Figure 1 from the Ban Suan Heritage Company in Yan Ta Khao District, Trang Province, Thailand, in June 2023 (at coordinates 7°23′12″N 99°40′0″E). Voucher specimens (PCMU 0024378) have been deposited at the Herbarium of the Department of Pharmaceutical Sciences, Faculty of Pharmacy, Chiang Mai University.
Trang peppercorn extraction
Seeds of BP, RP, and WP Trang peppercorn samples were extracted using 100 mL of DI water. A 20 g Trang peppercorn sample was homogenized and soaked for 15 min. Subsequently, it underwent extraction utilizing the MAE technique, employing two different conditions: microwave power settings of 500 W for 30 sec and 300 W for 50 sec, according to the method of (Maneewan & Khonsarn, 2018). The extraction procedure was repeated three times, with each round being allowed to rest for the same duration as the microwave exposure time. The extracts that were filtered and collected were evaporated until they were completely desiccated. The extraction yields for each sample are presented in Table 1.
Phytochemical contents
Determination phenolic content
The quantification of total phenolic compounds was carried out according to the method of (Damsud, Tedphum, Sukkrong, & Lila, 2021). This involved the preparation of 125 µL of Trang peppercorn extract, which was placed in a 96-well plate. Subsequently, 100 µL of 10% Folin-Ciocalteu reagent were added, followed by the addition of 20 µL of 7% Na2CO3 after a 5 min duration. The resulting mixture was thoroughly shaken and then left in a dark area at room temperature for 60 min. After the incubation time, the absorbance was determined using a spectrophotometer (SpectroStar Nano, Germany) at a wavelength of 765 nm. The total amount of phenolic compounds was calculated by comparing it with the calibration curve of gallic acid by expressing the value in milligrams of gallic acid per gram of extract (mg GAE/g).
Determination of flavonoid content
The measurement of flavonoids was performed using the aluminum chloride colorimetric test previously described by (Damsud et al., 2021). For the investigation, 0.5 mL of Trang peppercorn extract was mixed with a test tube containing 10% aluminum chloride, 0.15 mL of potassium acetate, and 0.65 mL of distilled water. After thorough mixing, the absorbance of resulting solution was monitored at 510 nm using spectrometer (BMG Labtech, Germany). The total flavonoid content was calculated in milligrams of quercetin per gram of extract (mg QE/g extract).
Determination of piperine
The determination of piperine content was modified from the method described by (Santosh, Shaila, Rajyalakshmi, & Rao, 2005). The Trang peppercorn extract was first filtered using a 0.45-micron filter paper. Subsequently, a volume of 20 µL from the filtered solution was introduced into the high-performance liquid chromatography (HPLC) system for analysis. The HPLC technique used an HITACHI LaChrom C18 column with dimensions of 250 x 4.6 mm I.D. The mobile phase consisted of 48% AcCN/H2O with 1% acetic acid, and the system operated at a flow rate of 1.5 mL/min. The light absorbance at a wavelength of 343 nm was evaluated to quantify the piperine concentration in the pepper samples by comparing them with a reference solution of piperine.
Determination of fatty acid
Gas chromatography was employed to determine the composition of fatty acids following an in-house method, TE-CH-208, which adhered to AOAC (2019) 996.06 (Piseskul et al., 2023).
α-Glucosidase inhibitory activity
Barker’s yeast α-glucosidase inhibitory activity
The assay was conducted following a slightly modified method based on (Damsud, Adisakwattana, & Phuwapraisirisan, 2013). Briefly, 10 µL of the Trang peppercorn extract dissolved in DMSO (at concentrations of 0.1, 0.25, 0.5, and 10 mg/mL) was combined with 0.1 M phosphate buffer (pH 6.9, 50 µL) containing α-glucosidase (0.1 U/mL, 40 µL). After a preincubation for 10 min at 37 °C, a 1 mM pNPG solution (50 µL) made using the same buffer was added. The reaction mixture was further incubated for 20 minutes and stopped by adding 100 µL of 1 M Na2CO3. The enzymatic hydrolysis of the pNPG was assessed by measuring the amount of p-nitrophenol released into the reaction mixture using a microplate reader (BMG Labtech, Germany) at 405 nm. The experiment was conducted in duplicate. The percentage inhibition and IC50 of activity were determined as follows:
% inhibition = [(A0-A1)/A0]× 100
Where: A0 is the absorbance without the sample; A1 is the absorbance with the sample.
Rat intestinal α-glucosidase inhibitory activity assay
The assessment of α-glucosidase inhibitory activity was conducted following a previously established procedure with minor modifications (Damsud et al., 2021). A raw enzyme solution sourced from rat intestinal acetone powder (Sigma-Aldrich) was employed as the origin of maltase and sucrase. To prepare this solution, 1.0 g of rat intestinal acetone powder was reconstituted in 30 mL of a 0.9% NaCl solution. After centrifugation (12,000×g for 30 min), the resulting supernatant was utilized for the assay. A 10 µL of the Trang peppercorn extract (at concentrations of 0.1, 0.25, 0.5, and 10 mg/mL in DMSO) was pre-incubated with the crude enzyme solution (as maltase, 20 µL; as sucrase, 20 µL, respectively) at 37 ºC for 10 min. Following this, a solution containing maltose (0.58 mM, 20 µL) and sucrose (20 mM, 20 µL) in 0.1 M phosphate buffer (pH 6.9) was introduced into the reaction mixture and allowed to incubate at 37 ºC for 40 min. The amount of glucose released from the reaction mixture was determined using the glucose oxidase method along with a glucose kit (Human, Magdeburg, Germany). The absorbance was measured at 540 nm (BMG Labtech, Germany), and acarbose was utilized as a positive control. The experiment was conducted in duplicate. The percentage inhibition and IC50 of activity were calculated employing a similar expression as described above.
Immune-stimulation assay
Determination of NO production
The Trang peppercorn extract was evaluated for NO production by macrophage J774A.1 cells utilizing Griess's method (Harun, WANW, & R, 2021). Initially, J774A.1 cells were cultured in a 96-well plate with a volume of 150 µL/well at 37 ºC in an atmosphere of 5% CO2 and 95% air. Subsequently, Trang pepper extract volumes ranging from 1.00 to 200 µg/mL were added, followed by a 24-hour incubation period. DMEM and LPS were used as the positive and negative controls, respectively.
Determination of cell viability
The effect of the Trang peppercorn extract on cell viability was assessed using the resazurin reduction assay (Harun et al., 2021). The procedure involved removing the supernatant from the treated cells and subsequently adding 100 µL of fresh, complete DMEM medium containing 50 µL of resazurin. The mixture was then incubated for 2 hours at 37 oC in an atmosphere of 5% CO2 and 95% air. The reduction of resazurin to resorufin by viable cells was determined by measuring the absorbance at 570 and 600 nm using a microplate reader (BMG Labtech, Germany). The percentage of viable cells was determined by comparing them with the control using the following formula:
% viability =
[(OD570-OD600)sample/(OD570-OD600)DEME]× 100
Determination of phagocytic activity
The phagocytic activity of macrophage J774A.1 cells was assessed via the zymosan NBT reduction assay (Harun et al., 2021). The J77A.1 cells were incubated at 150 µL/well at 37 ºC, within an atmosphere of 5% CO2 and 95% air. The cells were treated with Trang peppercorn extract ranging from 1.00 to 200 µg/mL, followed by a 24-hour incubation period. Negative control samples were treated with incomplete DMEM, while positive controls were administered 100 ng/mL LPS. Following this, the supernatant was carefully removed, and the cells were washed twice with DMEM. Zymosan (800 µg/mL) and NBT (600 µg/mL) were added to each well, and the cells were further incubated at 37 ºC for 1 hour. The cells were then washed three times with 200 µL of 2 M potassium hydroxide and 140 µL of DMSO. The NBT reduction was determined by measuring the absorbance at 570 nm using a microplate reader (BMG Labtech, Germany). The percentage of phagocytosis stimulation was determined using the given formula.
% phagocytosis stimulation =
[(OD570-OD570)control/(OD600)control]× 100
Statistical analysis
The results are displayed as the mean ± standard deviation (S.D.) for three independent experiments. Significant results were detected using a p-value level of less than 0.05 and 0.01 for the one-way analysis of variance (ANOVA). The statistical analysis was performed using SPSS version 20.0 (IBM SPSS, IBM Corp., Armonk, New York).
Table 1
Fatty acid composition of the MAE extracts from Trang peppercorn.
Table 2
Extraction yield and total phenolics and flavonoids contents of the MAE extracts from Trang peppercorn.
Table 3
α-Glucosidase inhibition of the MAE extracts from Trang peppercorn.
RESULTS AND DISCUSSION
Effects of MAE conditions and peppercorn type on phytochemical content
We studied the effects of the MAE technique, which we employed to prepare BP, RP, and WP extracts of Trang peppercorn under different conditions. The examination of the fatty acid composition in the three types of peppercorn samples revealed the presence of both saturated, monounsaturated, and polyunsaturated fatty acids. It was observed that using a microwave with a power of 500 W for 30 sec resulted in a higher fatty acid value than using a power of 300 W for 50 sec, showing statistical significance at p < 0.05. The group's extraction results at 300 W for 50 sec and 500 W for 30 sec revealed significant differences in the amounts of palmitic acid, oleic acid, and linoleic acid, which were significantly different at the confidence level of p < 0.05 (Table 1) The phytochemical content analysis clearly showed that using a microwave operating at 500 W for 30 sec was the most suitable extraction condition. This condition resulted in extraction yields (%) of 11.58±0.65 for BP, 17.42±0.65 for RP, and 7.54±0.03 for WP. The total phenolic content values were 13.92±0.01 mg GAE/g for BP, 105.92±0.01 for RP, and 42.30±0.01 for WP. The total flavonoid content measured (mg QE/g) was 47.20±0.04 for BP, 61.80±0.05 for RP, and 37.53±0.03 for WP, respectively (p < 0.05) (Table 2). Additionally, the piperine content (%) in BP was found to be 3.57±0.20 in was 2.64±0.34 and in 2.82±0.30 in RP and WP, respectively (p < 0.05). If the extraction results within the group at 300 W for 50 sec and 500 W for 30 sec are considered, it was found that extraction yields (%), TPC, and TFC values were significantly different at the confidence level p < 0.05, while piperine (%) showed no significant difference within the same group (p < 0.05) (Table 2). The investigation focused on the suitable conditions for microwaving to extract substances from medicinal plants. It was determined that the optimal conditions for this extraction encompassed a power range of 250–300 W and a duration lasting 70–80 sec (Özbek, Yanık, Fadıloğlu, & Göğüş, 2020). Moreover, the extraction efficiency could be impacted by the ratio of medicinal plants to the volume of solvent or the specific type of solvent utilized. Throughout the extraction process, minimal microwave radiation was applied to the extract sample, and the power was set lower, prolonging the exposure time to the waves. This adjustment aimed to prevent potential alterations in the chemical structure of the medicinal plant's active ingredients, which might have occurred with higher power settings (Bagade & Patil, 2021; Silva et al., 2022). Microwave-assisted extraction of piperine using nonpolar solvents was previously reported by (Raman & Gaikar, 2002). This technique provided rapid extraction and afforded higher yield of the desired target without degradation. Nevertheless, such solvents are capable of posing toxicity risks and exhibit limitations in their applicability across various applications.
α-glucosidase inhibitory activity
Another method utilized for regulating sugar levels involved investigating the inhibition of carbohydrate absorption within the digestive system, specifically in the intestines, to suppress α-glucosidase activity. This method was considered one of the most effective approaches to reducing blood sugar levels after meals. Thus, the focus of this study was to examine the inhibition of α-glucosidase obtained from yeast and the rat small intestine. This investigation entailed using a crude enzyme extract containing maltase and sucrase, featuring a mechanism of action akin to that observed in the human body. The study revealed that BP and WP extract, through MAE extraction of 500 W for 30 sec, exhibited the most effective inhibition of yeast and maltase α-glucosidase, presenting an IC50 value of 2.09±0.05 and 1.70±0.05mg/mL respectively, similar to acarbose, which also displayed a value of 2.99±0.07 and 0.49±0.05 mg/mL respectively. Furthermore, the IC50 value for RP extract, utilizing a microwave power of 500 W for 30 sec, was 3.02±0.04 mg/mL, demonstrating superior efficiency in inhibiting sucrase in rat intestinal α-glucosidases. These values surpassed those of acarbose, which demonstrated IC50 values of 0.81±0.15 mg/mL (Table 3 ). Studies on active substances derived from plants and herbs have identified numerous groups of compounds with the potential to inhibit α-glucosidase. However, the effectiveness of these inhibitors can vary, influenced by both the types of substances and the specific enzyme involved. Flavonoids, alkaloids, and phenolic compounds have been extensively studied. Effectiveness in inhibiting α-glucosidase enzymes derived from both baker's yeast and the small intestine of rats, especially maltase and sucrase, has been demonstrated by this group of substances. Trang peppercorn contain these compounds. For example, the study conducted by (Temrangsee, Itharat, Sattaponpan, & Pipatrattanaseree, 2019). focused on piperine within the Piperaceae family, which was extracted using a 95% ethanol solvent and under gastric stimulation conditions. This study successfully demonstrated the inhibition of yeast α-glucosidase, revealing IC50 values of 387.72±3.15 and 241.02±0.60 µg/mL, respectively. Additionally, studies have been conducted on piperine and docking with α-glucosidase, demonstrating their effectiveness. Researchers discovered its effectiveness; when employed as ligands, it demonstrated an energy reduction of -6.7 to -8.7 kcal. Such studies are useful in developing drugs that effectively lower postprandial blood sugar levels (Tuli et al., 2021). Flavonoids found in pepper, such as catechin, myricetin, quercetin, and lutein, were found to be effective in inhibiting α-glucosidase from yeast and sucrase in the rat's small intestine. Additionally, similar inhibitory efficiency was demonstrated by phenolic compounds (Ashokkumar, Murugan, Dhanya, Pandian, & Warkentin, 2021; Lu et al., 2019; Magaña-Barajas et al., 2021).
Figure 2
Effect of various concentrations of the MAE extracts of (A) Black pepper (BP); (B) Red pepper (RP); (C) White pepper (WP) on NO production in macrophage J774A.1cells, with a positive control (100 ng/mL LPS) for a duration of 24 hours. The data, representing the mean ± SD from 3 independent experiments (n=3); *, ** =significant difference with the untreated control (media) at p<0.05 and p<0.01, respectively.
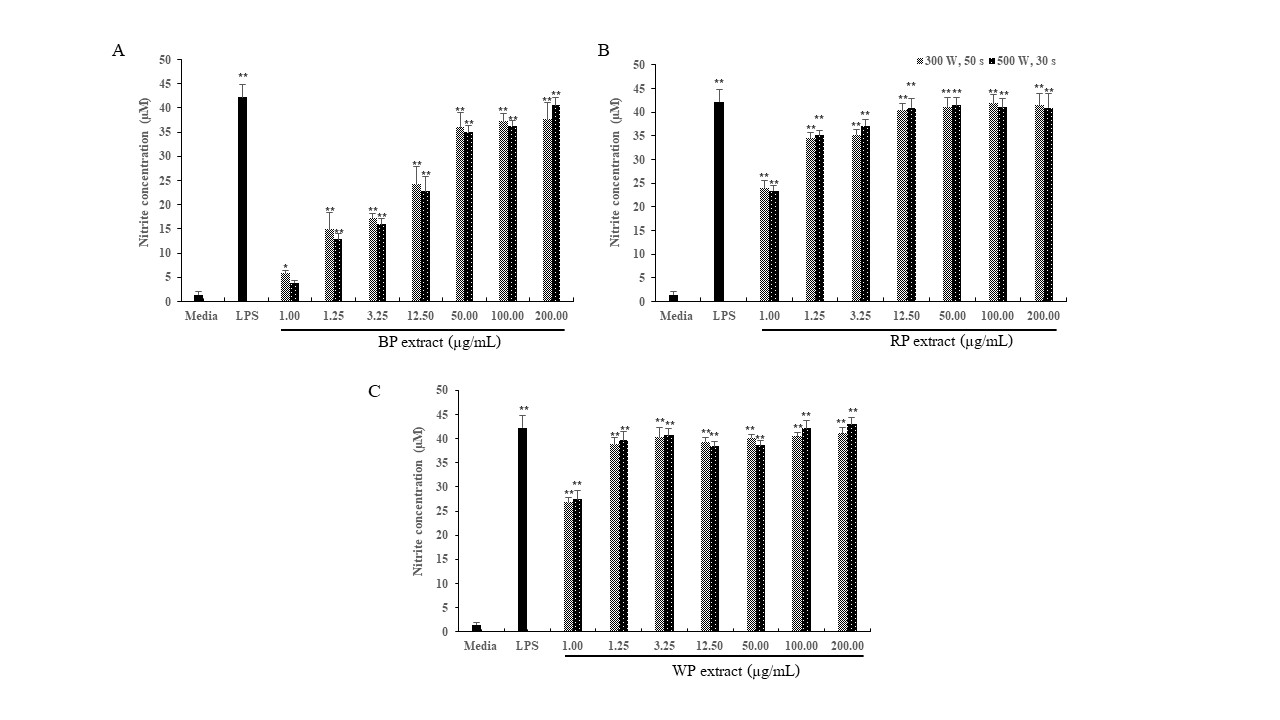
Immune-stimulation assay
Immunomodulators specifically target a range of mediators, immune system cells, and macrophages. Macrophages, being involved in both innate and adaptive immunological responses, are highly susceptible to immune enhancers. The oxygen-dependent system produces nitric oxide (NO) to destroy internalized pathogens during phagocytosis, hence making a substantial contribution to the elimination of infections. Furthermore, NO serves as a commonly used indicator for identifying macrophage activity. Consequently, it serves as the main criterion for evaluating the capacity of Trang peppercorn MAE extracts to activate macrophage cells. Upon synthesis, NO rapidly undergoes oxidation, leading to the creation of nitrite. The quantity of nitrite is directly proportional to the quantity of NO present. The quantity of nitrite was determined as a stable byproduct of NO using Griess' reagent. MAE of three types of Trang peppercorn extracts—BP, RP, and WP—led to more NO being made in macrophage J774A.1 cells. This effect was seen when employing MAE at power levels of 300 W for 50 sec and 500 W for 30 sec, with a concentration of 1.00 µg/mL. In addition, this extract demonstrated a substantial rise in the synthesis of NO in a way that was dependent on the concentration, ranging from 1.25 to 200.00 µg/mL (Figure 2 ). The effect on cell survival was tested using a resazurin reduction assay to find the best amount of Trang pepper extracts and to see if they could affect cell survival. The mitochondrial reductase enzyme has the ability to transform the blue hue of resazurin into the pink hue of resorufin inside living cells. Cell viability was assessed during a 24-hour incubation period with different doses of Trang pepper extracts or 100 µg/mL LPS. The findings indicated that Trang pepper extracts did not have any impact on the viability of J774A.1 cells at all of the concentrations examined (Figure 3 ).
Figure 4
Effect of theMAE extracts of (A) Black pepper (BP); (B) Red pepper (RP); (C)White pepper (WP) derived from Trang peppercorn on the phagocytic activity ofmacrophage J774A.1 cells. The data are presented as mean ± SD obtained from 3independent experiments (n=3). *, ** = significant difference with theuntreated control (media) at p<0.05 and p<0.01,respectively.
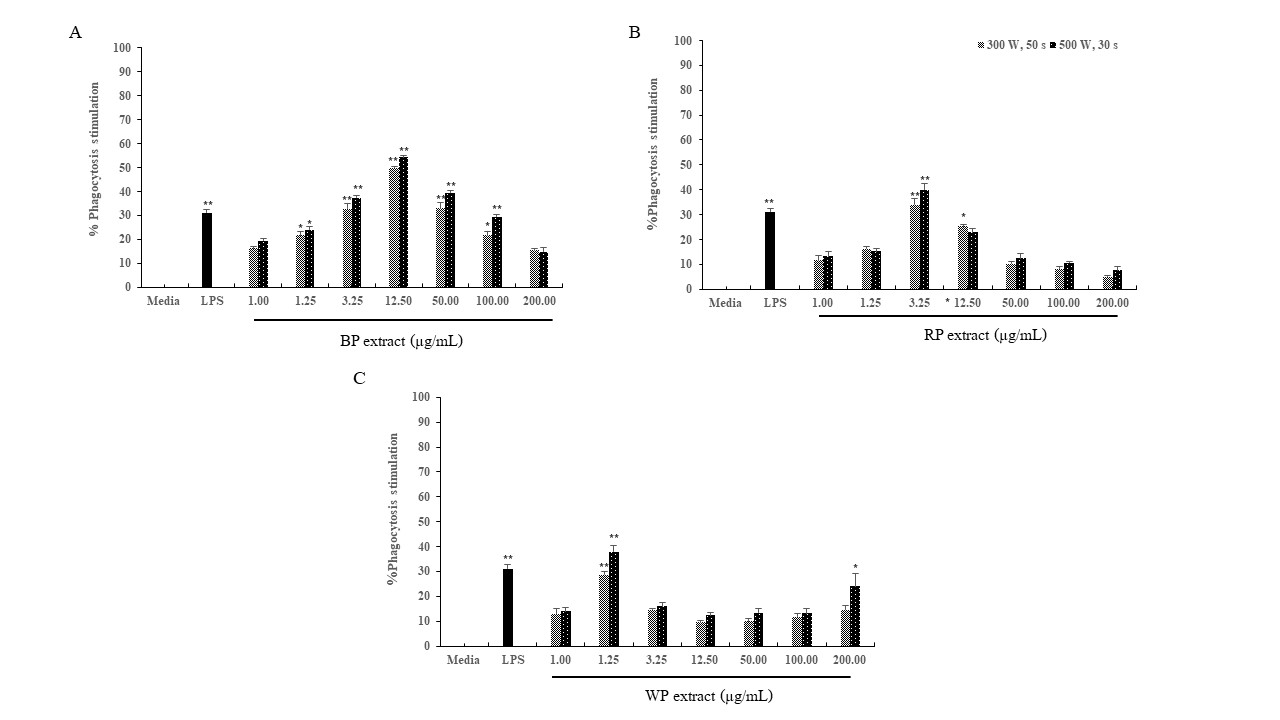
Hence, the Trang pepper extract does not exhibit any cytotoxicity, and all concentrations of the extract were deemed appropriate for experimentation. Macrophages are the main phagocytes in tissues that have the ability to ingest and destroy foreign particles or pathogens by engulfing them via phagocytosis. The phagocytic activity was evaluated in this study utilizing a zymosan NBT reduction assay. Respiratory bursts take place during phagocytosis, leading to an elevation in the synthesis of hydrogen peroxide (H2O2) and superoxide free radicals. The research on Trang pepper showed a greater degree of phagocytosis stimulation when extracted utilizing the MAE approach at a power of 500 W for 30 sec, as opposed to extraction at 300 W for 50 sec. Both BP and RP extracts exhibited macrophage-stimulating properties at concentrations ranging from 1.25 to 100 µg/mL and 3.25 to 12.50 µg/mL, respectively. However, the degree of stimulation decreased as the concentration rose. Unlike the positive control LPS at a concentration of 100 ng/mL, the WP extract was capable of activating macrophages at concentrations of 1.00 and 200 µg/mL (Figure 4 ). Piperine, the main alkaloid of P. nigrum, was shown in many previous studies to have an inhibitory effect on macrophage activity when added to an extract. Piperine has been thoroughly studied and demonstrated to have a variety of pharmacological actions, such as its inhibition of macrophage activation, which has an anti-inflammatory impact (Pei et al., 2020).
It inhibited macrophages from releasing pro-inflammatory cytokines and NO. It is less probable that piperine is the source of the stimulatory effects of pepper water extract on macrophages (Pal, Begum, Basha, Araya, & Fujimoto, 2023; Yu, Liu, Yu, Changyong, & Yang, 2020). The polysaccharide-rich found in peppercorn may be the source of the stimulatory action (Duan et al., 2022). A previous study indicates the immunostimulatory effects of the pepper water extract used in this investigation. Pepper water extract stimulated mouse peritoneal macrophages, prompting them to produce TNF-α, IL-6, and NO. In addition, it stimulated the secretion of IFN-γ and splenocyte proliferation. Furthermore, it inhibited splenocytes from producing IL-10 and IL-4, both inhibitory cytokines (Neha & Joshi, 2020). Linoleic acid, which is a type of fatty acid, is polyunsaturated and is found in high amounts in Trang pepper. It has been reported to be effective in stimulating Staphylocccus aureus infected J774A.1 macrophages (Yan et al., 2022). In addition, the fatty acid linoleic acid, which is produced by welding cis-9, trans-11, and trans-10, cis-12 CLA, was found to increase the creation and distribution of white blood cells and reduce (TNF-α) and interleukin 6 (IL-6) occurrence of necrosis of cancer cells. Moreover, there was increased production of IL-2 in the mice (Yang, Zhang, & Zhou, 2019).
CONCLUSION
The present study showed that MAE technique facilitates preparing Trang peppercorn extracts (BP, RP, and WP). Extracting the Trang peppercorn with microwave power of 500 W for 30 sec provided more powerful extraction efficiency, which was demonstrated by higher phytochemical contents obtained including stronger inhibition against α-glucosidases and enhanced immune response. The use of water as a solvent in this study also provided alternative methodology to afford piperine-enriched extract that can be further applied in formulating herbal medicine without toxicity caused by solvent residue. This research could result in the discovery of a combination therapy for people with diabetic complications whose high blood sugar levels will possibly decrease the immune system. It could additionally open up possibilities for future research and development as a potential medication for the medical management of diabetes.