Introduction
Excessive free radical production and lipid peroxidation in vivo are known to cause various diseases such as cancer, diabetes mellitus, inflammation, several degenerative diseases, and sickle cell disease (SCD) (Bolarinwa et al., 2024; Chaudhary et al., 2023; Tumilaar, Hardianto, Dohi, & Kurnia, 2024; Vona et al., 2021). Sickle cell disease or sickle cell anemia, is a genetic desorder induced by mutations in the genes responsible for synthesis of hemoglobin. Approximately 300,000 babies are born worldwide each year with severe forms of this hemoglobinopathy (Gbolo et al., 2023; Vona et al., 2021). It remains the leading cause of stroke in children, with cerebral vasculopathy being the most serious complication in pediatric patients (Abdel-Hadi et al., 2023; Kirkham & Lagunju, 2021; Marks et al., 2018).
Studies have demonstrated that increased oxidative stress and an abnormal oxidant/antioxidant balance play a significant role in the pathophysiology of SCD (Antwi-Boasiako et al., 2019). Managing SCD is particularly challenging in developing countries such as the Democratic Republic of the Congo (DRC), where public healthcare is lacking and medical expenses are generally borne by patients' families or employers. The DRC's population includes approximately 25% with AS genotypes and nearly 2% with SS genotypes (Gbolo et al., 2023; Tshilolo et al., 2009). For this population, access to existing drug treatments like Hydroxyurea, L-glutamine, Crizanlizumab, and Voxelotor is limited by availability or cost (Abdel-Hadi et al., 2023).
Incorporating antioxidant-rich foods and fruits into the diets of SCD patients as a treatment strategy to boost immunity and naturally combat the opportunistic infections that increase free radical release, responsible for cell apoptosis and shortened cell lifespan, is essential (Ifeanyi, Stella, & Favour, 2018). Developing phytomedicine based on traditional pharmacopoeia knowledge is emerging as a viable solution for African countries like the DRC. The World Health Organization (WHO) estimates that nearly 80% of the African population relies on traditional medicine for primary healthcare (WHO, 2022).
Justicia secunda Vahl (Acanthaceae), a native tropical herbaceous plant from South America, now cultivated in other tropical or subtropical African countries like the DRC, was selected for this study. Initially an ornamental plant, its leaves have been used to treat various ailments such as anemia and hypertension and are now widely used for numerous medicinal applications (Anyasor, Okanlawon, & Ogunbiyi, 2019; Mea, Ekissi, Abo, & Kahou, 2017; Ogunbamowo, Olaniyi, Awotedu, & Lawal, 2020). In the DRC, this species has proven effective in managing anemia and SCD in traditional Congolese medicine (Mpiana et al., 2010; Mpiana, Ngbolua, & Tshibangu, 2016).
Documenting and preserving this traditional knowledge is crucial, as it can contribute to developing modern medicines by identifying lead compounds, examining drug-like activity, and exploring physicochemical, biochemical, pharmacokinetic, and toxicological characteristics, thus significantly reducing costs, especially for low-income countries. Based on chemical composition data of the chosen species (Kitadi et al., 2019), flavonoids were targeted due to their potent free radical scavenging, antioxidant (Hassanpour & Doroudi, 2023; Ifeanyi et al., 2018), anti-inflammatory (Al-Khayri et al., 2022; Ginwala, Bhavsar, Chigbu, Jain, & Khan, 2019; Shamsudin et al., 2022), anticarcinogenic (Kopustinskiene, Jakstas, Savickas, & Bernatoniene, 2020; Luna, Ferreira, Casseb, & Oliveira, 2023; Mir et al., 2024), antidepressant (Guillén-Ruiz, Bernal-Morales, Limón-Vázquez, Olmos-Vázquez, & Rodríguez-Landa, 2024; Pannu, Sharma, Thakur, & Goyal, 2021; Rodríguez-Landa, German-Ponciano, Puga-Olguín, & Olmos-Vázquez, 2022), and antisickling (Adeniyi et al., 2022; Lukyamuzi, Oweta, Dominguez, Betancourt, & Rodríguez, 2019; Mohamed, Dayem, Shamy, Sakhawy, & Gedaily, 2023) properties. While the antioxidant properties of flavonoids have been the main focus of recent studies, their anti-sickling properties are less explored (Lukyamuzi et al., 2019).
To scientifically validate traditional medicine data and attribute this activity to a known metabolite, this study aims to conduct a phytochemical screening of crude flavonoids extracted from Justicia secunda Vahl from the DRC using LC-ESI/MS/MS and to assess their anti-sickling activity.
Materials and methods
Plant material
Mature leaves of Justicia secunda, untreated with insecticides or fungicides, were collected between April and May 2018 in the N'djili Brasserie area of Kinshasa, DRC (Latitude: South 004°28'24.92'', Longitude: East 015°21'2.80'', Altitude: 287.6 m; mean annual temperature: 25 ± 0.4 °C, mean annual precipitation: 127.3 ± 53.7 mm). The leaves were washed with water to remove soil particles, shade-dried at room temperature for three weeks, and then pulverized to a fine powder (< 5 mm) using a local mill. Professor Félicien Lukoki of the Department of Biology at the University of Kinshasa conducted the plant identification, confirming the plants as J. secunda Vahl (Herbarium MNHN-P-P00719831).
Blood samples
The blood sample used in this study was provided by the “Centre de Médecine Mixte et d’Anémie SS” in Kalamu, Kinshasa, DRC. None of the patients had recently undergone a transfusion with Hb AA blood. All antisickling experiments were conducted using freshly collected blood, which was then stored at ± 4 °C in a refrigerator. Red cell pellets were obtained by centrifuging (1500 g, 10 min) 0.5 mL of SS blood, washing the pellets three times with 9‰ NaCl in a 1:10 (v/v) ratio, centrifuging (1500 g, 10 min) after each wash, and finally resuspending the pellets in 4 mL of 9‰ NaCl. This study was conducted under ethical approval No.: ESP/CE/237/2019.
Chemicals
Heptane and methanol, used during sample extraction, were purchased from Chem-Lab and were of analytical grade. Methanol, used for isolation, purification, and HPLC-MSMS experiments, was also purchased from Chem-Lab and was of HPLC grade. Additionally, formic acid (>98% purity), ethyl acetate (98% purity), heptane (98% purity), isopropanol (98% purity), and methylethylketone (98% purity) were used. Ultrapure water was obtained using a Purelab Flex system (Veolia).
For structural characterization, standard flavonoids were used, including apigenin (Sigma-Aldrich, >97%), apigetrin (Ph. Eur. Reference Standard, 93%), cynaroside (Extrasynthese, >98%), kaempferol (Sigma-Aldrich, 90%), and luteolin (Sigma-Aldrich, 98%). Rutin trihydrate, purchased from Sigma-Aldrich (>95% purity), was used for quantification.
Preparation of the methanolic extract of J. secunda
The dry methanolic extract (drug-extract ratio, DER, 500/21.14) was prepared by first percolating 500 grams of J. secunda powder with heptane (ratio 1:10, w/v) for 72 hours to remove lipids. After filtration and drying the powder for 6 hours in a laminar flow hood, the residue was extracted again with methanol under the same conditions. Following filtration, the extract was concentrated by vacuum evaporation (Büchi R-210) at 40 °C. The resulting dry methanolic extract was stored in glass containers at approximately 4 °C in a refrigerator.
Isolation and purification of flavonoids
Isolation and purification of flavonoids were performed as described by Gbolo et al. (2022). The experimental procedure for these methods were provided as supplementary information.
LC-MS/MS analysis
Sub-fractions FP2-FP9 (0.0586 g) were diluted to a 1/100 concentration in methanol for mass spectrometry analysis. HPLC-MS and HPLC-MS/MS analyses were performed in negative mode using a Waters Synapt G2-Si mass spectrometer equipment (Waters, Manchester, UK) fitted with an electrospray ion source, and paired with a Waters Acquity UPLC H-Class system (Waters, Manchester, UK). The chromatographic separation was performed on a reversed-phase Phenomenex Kinetex C18 EVO column (150 × 2.1 mm i.d., 5 µm particle size). A binary gradient was applied at a flow rate of 0.25 mL/min, with the mobile phases consisting of water + 0.1% formic acid (solvent A) and methanol (solvent B). The gradient program was as follows: A = 90%, B = 10% at 0 min; A = 70%, B = 30% at 6 min; A = 65%, B = 35% at 11 min; A = 50%, B = 50% at 18 min; A = 10%, B = 90% at 23 min; A = 0%, B = 100% at 25 min; A = 0%, B = 100% at 27 min; and A = 90%, B = 10% at 30 min. The column temperature was maintained at 40°C. In the mass spectrometer, parameters such the capillary voltage, the cone voltage, the source temperature, and the desolvation temperature were respectively set to 2.5 kV, 40 V, 120°C and 300°C.
HPLC-MS and HPLC-MSMS experiments in positive mode were conducted using a Waters Q-ToF US mass spectrometer equipment (electrospray ion source), coupled with a Waters Alliance 2695 HPLC system (Waters, Manchester, UK). This experiment was carried out as described in negative mode except that the capillary voltage was set to 3.1 kV and the cone voltage to 30 V.
For quantification, samples were spiked with a known concentration of rutin and analyzed by HPLC-MS in triplicate using the Waters Synapt G2-Si mass spectrometer. The total flavonoid concentration was determined by comparing the flavonoid peak areas to the rutin response, expressed in rutin equivalents. To ensure that the recorded intensity was proportional to the concentration, a calibration curve was established with rutin.
In vitro antisickling activities
Sample Preparation
A 9 ‰ normal saline solution (NaCl 9 ‰) was used to dilute whole blood at a 1:10 (v/v) ratio. Sodium metabisulfite 2% (Na2S2O5 2%) served as the deoxygenating agent. Crude flavonoid extracts from J. secunda were the experimental samples. Disodium cromoglycate acted as the positive control, while NaCl 9 ‰ served as both the negative control for all experiments and the blank in spectrophotometric analyses.
Anti-sickling properties
Anti-sickling properties of crude flavonoid extracts from J. secunda, were evaluated by estimation of some parameters including (a) the Hemolytic assay as described byHaddad, Laurens, and Lacaille-Dubois (2004) then modified and adapted by Gbolo et al. (2022), (b) Polymerization inhibition were conducted using the original method described byNoguchi and Schechter (1978) and completed byGbolo et al. (2023) and Mishra, Sonter, Dwivedi, and Singh (2022) (c) Hypoxia and sickling, (d) Emmel test, (e) Determination of the Minimum Reversibility Concentrations (MRC), (f) oxidation inhibition and (f) osmotic fragility test was conducted following the method described by Gbolo et al. (2023, 2022). The detailed procedure of each method are provided as supplementary information.
Results and Discussion
Flavonoid fractionation and purification
Figure 2 shows the HPTLC profile of fractions of the crude extract of Justicia secunda.
Figure 2
HPTLC profile of fractions of the crude extract of Justicia secunda. Legends : F1: Fractions from the 1st fractionation of the crude extract; F2: Fractions from the fractionation of the polarfraction: (a) Chromatogram examined under UV-366 nm, (b) Chromatogram examined under visible light. Mobile phase : AcOEt-MEK-AF-EAU60/30/5/5 v/v/v; derivatization with NP (heating the plate at 100 °C for 3 min)and PEG-400.
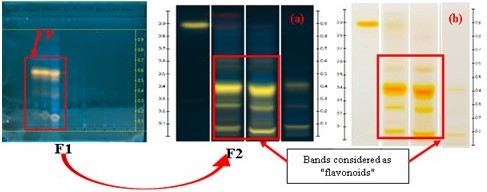
Table 1 gives the flavonoids content in the methanolic extract ofJusticia secunda leaves powder.
Table 1
Flavonoid content expressed as Q-equivalent in Justicia secunda sample by HPTLC.
Yellow bands with an Rf value of 0.41 were identified as flavonoids, and their density was quantified as Quercetin equivalents based on their area under the curve (AUC). Qualitative analysis using HPTLC confirmed the presence of flavonoids, as indicated by the yellow spots observed under UV light at 366 nm upon reaction with Neu's reagent. This finding aligns with previous studies (Gbolo et al., 2017). Both the crude methanolic extracts and the various fractions exhibited these yellow spots, validating the presence of flavonoids (Lawag et al., 2022; Liu et al., 2018; Magnini et al., 2020) (Lawag, Sostaric, Lim, Hammer, & Locher, 2022; Magnini, Hilou, Millogo-Koné, Pagès, & Davin-Regli, 2020). Quantitative analysis further supports these observations, demonstrating that the extracts contain significant amounts of flavonoids (Table 1).
LC-MS(MS analysis of flavonoids
LC-MSMS analysis was pursued for further structural characterization of J. secunda purified extracts. This combination is especially powerful and helpful; it allowed us to identify 12 flavonoids in Justicia secunda Vahl from DRC (Table 2 ). Those flavonoids are glycosides of three distinct aglycones, one flavonol (methoxy-kaempferol) and two flavones (luteolin and apigenin) (Figure 3 ).
HPLC-MS experiments achieved a good separation of the flavonoids and showed the presence of several isomers (Figure 4 ).
Figure 4
Chromatogram from the HPLC-MS analysis of the purified J. secunda extract (Waters Acquity UPLC H-Class and Waters SynaptG2-Si).
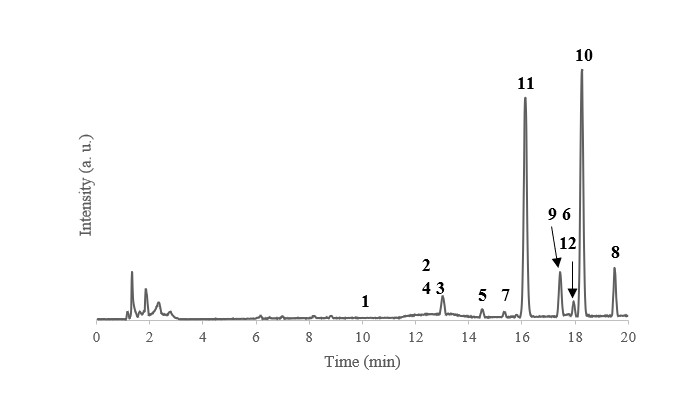
In this study, flavonoids were primarily analyzed in negative mode due to the susceptibility of protonated flavonoid ions to in-source dissociation, which complicates their characterization. CID spectra for standard flavonoids, including those from our lab and referenced in Cuyckens and Claeys (2004), were used to interpret the data.
At low collision energies, deprotonated ions typically lose their sugar chains. For O-glycosylated flavonoids, the glycosidic linkage between the aglycone and the sugar chain is cleaved, resulting in the loss of the entire chain in a single step. This pattern was observed in the flavonoids analyzed (refer to Supplementary Information for CID spectra), allowing us to identify them as O-glycosylated rather than C-glycosylated. C-glycosylated flavonoids, characterized by stronger C-C bonds, would exhibit a cross-cleavage of the saccharidic units.
Di- and tri-O-glycosylated flavonoids showed only one sugar loss in negative mode, indicating that the monosaccharidic units are linked to the flavonoid aglycone at a single position.
At higher collision energies, aglycones undergo fragmentation, resulting in the loss of small molecules (CO, CO2, H2O) and cleavages of the C-ring through a retro-Diels-Alder (RDA) mechanism, which helps identify the positions of substituents on the A and B rings (see Figure 3 ). Methylated aglycones also display losses of methyl radicals as the first fragmentation products (Silva & Lopes, 2015).
To determine the nature and sequence of monosaccharidic units in sugar chains, positive mode analysis was employed. Protonated ions break glycosidic bonds at low collision energies, providing insight into the sugar chain composition.
Based on this comprehensive data, the proposed names for the detected flavonoids are listed in Table 3. Mass accuracy was determined using HPLC-MS spectra from the Waters Synapt G2-Si, with corresponding CID spectra available in the Supplementary Information. Tables 2 and 3 detail the fragment ions and proposed names for the identified flavonoids in Justicia secunda Vahl.
Table 2
Fragment ions of each flavonoid ion in negative mode and in positive mode, depending on the collision energy.
Table 3
Proposed names for the identified flavonoids in Justicia secunda Vahl (DRC) purified extract, retention time, formula, m/z of corresponding deprotonated ions, mass accuracy and relative abundance.
In the present study, twelve flavonoid O-glycosides were identified in Justicia secunda, including one flavonol (kaempferide) and two flavones (luteolin and apigenin).
Table 2 shows that peaks (1), (3), (4), (7), (9), and (11) exhibited similar fragmentation patterns with losses at m/z 285, 284, 256, 227, 199, 175, 151, 133, and 107. The presence of a fragment at m/z 256 indicates that these compounds are flavone glycosides and contain luteolin. Based on chromatographic column behavior and CID spectrum data, peak (1) was identified as Luteolin-7-O-hexosylhexosylrhamnoside; compound (3) was identified as Luteolin-7-O-hexosylrhamnosylrhamnoside; and compounds (4), (7), (9), and (11) were assigned as Luteolin-7-O-hexosylrhamnoside, Luteolin-7-O-pentosylhexoside, Luteolin-7-O-pentosylrhamnoside, and Luteolin-7-O-dipentoside, respectively. These luteolin glycosides have been previously reported in this plant (Koffi et al., 2013).
Peaks (2) and (12) with [M-H]– ions at m/z 739 and 533 showed similar fragmentation behavior, yielding fragments at m/z 269, 159, 151, 117, and 107. According to Zhou et al., (2018) the fragment at m/z 269 suggesting the presence of apigenin. Therefore, compounds (2) and (12) were identified as Apigenin-7-O-hexosylhexosylrhamnoside and Apigenin-7-O-dipentoside, respectively.
Peaks (5) and (6) exhibited the same [M-H]– ion at m/z 593, with fragmentation producing peaks at m/z 299, 284, 255, 227, and 151. Similar fragmentation patterns were observed for peaks (8) and (10) with [M-H]– ions at m/z 577 and 563. The fragments at m/z 284 and 255 are characteristic of kaempferol glycosides. Consequently, compounds (5) and (6) were identified as Kaempferide-7-O-hexosylpentoside and Kaempferide-7-O-pentosylhexoside, while compounds (8) and (10) were identified as Kaempferide-7-O-pentosylrhamnoside and Kaempferide-7-O-dipentoside.
Quantitative analysis of the crude flavonoid extracted from J. secunda species from the DRC revealed that the luteolin family is the most predominant, constituting 52.40 ± 0.45% of the extract. This is followed by kaempferide at 43.47 ± 0.52%, and apigenin at 2.66 ± 0.12%. The high proportion of luteolin aligns with the qualitative HPTLC analysis, which identified a characteristic yellow spot for this compound upon reaction with Neu's reagent (Lawag et al., 2022).
Hemolytic assay
Table 4 describes the hemolytic effect of flavonoid on red blood cells.
Table 4
Test for hemolytic action of crude flavonoid extracts and sodium cromoglycate (n=3 biological replicates, Concentration= 1mg/mL, Time= 30 min).
The fragility of red blood cells (RBCs), characterized by repeated cycles of sickling and reversibility that can lead to hemolysis, is a notable feature of sickle cell disease. Plant compounds, such as saponins, may also contribute to hemolysis and potentially skew assay results.
As a primary indicator for selecting and confirming plant extracts for managing sickle cell disease, it is essential to evaluate their hemolytic activity. According to Gbolo et al. (2022), an extract is deemed acceptable if its hemolytic activity is ≤5%, to avoid introducing bias by altering RBC quantities during experimentation.
In this study, the crude flavonoid extracts from Justicia secunda demonstrated very low hemolytic activity, making them suitable for application to RBCs without introducing bias. The observed hemolysis due to disodium cromoglycate (2.1%) was considered negligible.
Reversibility assay
Figure 5 below shows phenotypic micrographs of red blood cells untreated and treated with crude extracts of J. secunda flavonoids or disodium cromoglycate.
Figure 5
Morphology of drepanocytes of SS blood (B), upon treatment with disodium cromoglycate (250 µg/mL, A) and crude flavonoids extracted from Justicia secunda, 125 µg/mL (Right) (60 min contact with sodium meta bisulphite inair-tight conditions; 500 X).
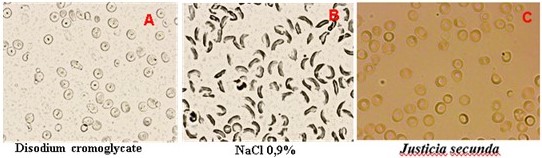
Figure 6 presents the reversibility rate of sickled red blood cell according to the crude flavonoids extracted from Justicia secunda concentration
Figure 6
Reversibility rate of sickled red blood cell according to concentration of crude flavonoids extracted from Justicia secunda (60 min contact with sodium meta bisulphite inair-tight conditions; MRC, minimum reversibility concentration; MRR, maximum reversibility rate). (Data from 3 biologicaltests in triplicate, Bars represent the mean ± SD.
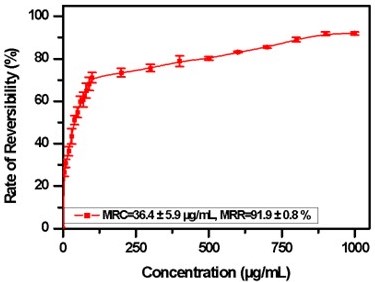
Sickle cell disease is a hemoglobinopathy characterized by the presence of hemoglobin S, posing a significant public health challenge in endemic regions (OMS, 2010). Despite extensive research and a comprehensive understanding of its pathophysiology, no specific treatment exists; management primarily focuses on symptomatic relief (Abdel-Hadi et al., 2023; Sall et al., 2016).
In the Democratic Republic of Congo, hydroxyurea is the primary treatment for sickle cell crises. However, this drug is not widely available and poses cytotoxic risks with prolonged use (Demontalembert & Tshilolo, 2007; Kitambala et al., 2021).
For the past 15 years, our research team has been investigating anti-sickle cell plants from the traditional Congolese pharmacopoeia (Mpiana et al., 2007). Our studies have attributed pharmacological activity to anthocyanins, phenolic acids, and triterpenic acids (Mpiana et al., 2007; Mpiana et al., 2008; Mpiana, Tshibangu, Ngbolua, Tshilanda, & Atibu, 2007; Mpiana, Tshibangu, Shetonde, & Ngbolua, 2007; Tshilanda et al., 2015; Tshilanda, Ngbolua, Tshibangu, Malumba, & Mpiana, 2016). However, the role of flavonoids, known for their free radical scavenging properties and potential to protect RBCs from oxidative damage, has not been explored (Hassanpour & Doroudi, 2023; Sall et al., 2016).
In this study, flavonoids extracted from Justicia secunda in the DRC demonstrated exceptional potential for inhibiting sickle cell formation, with a reversibility rate of 91.9 ± 0.8%. When compared to other compounds from the same plant, these flavonoids exhibit superior activity to anthocyanins extracts from DRC (Mpiana et al., 2010) and hydroalcoholic extracts (83.50 ± 2.3%) from Ivory Coast (Kplé et al., 2020). They also outperform phenylalanine (80.60 ± 2.33%) (Kplé et al., 2020) and hydroxyurea (49.5 ± 0.5%) (Mishra et al., 2022).
Figure 6 illustrates that the percentage of reversibility of sickled cells under hypoxic conditions increases positively with the concentration of crude flavonoid extracts from J. secunda, reaching a plateau known as the Maximum Reversibility Rate (MMR). Beyond this plateau, reversibility remains constant despite further increases in concentration. The Minimum Reversibility Concentration (MRC), defined as 36.4 ± 5.9 µg/mL, represents the extract concentration required to normalize 50% of the sickled cell population. MMR and MRC were determined using non-linear regression analysis.
Stabilization of erythrocyte membranes
Figure 7
Evolution of thehemolysis rate as a function of NaCl concentration (MCF: median corpuscular fragility)
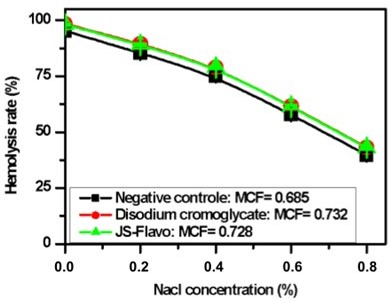
This graph shows that the rate of erythrocyte hemolysis decreases with increasing NaCl concentration; allowing to determine by interpolation the values of the Median Corpuscular Fragility (MCF), the NaCl concentration for which 50% of erythrocytes are hemolyzed.
Erythrocyte membrane stability, which we studied in sickle cell red blood cells under hypotonic NaCl conditions, appears higher upon treatment with sodium cromoglycate and flavonoid extracts from Justicia secunda. This indicates that flavonoids probably improve the hydration capacity of sickled cells, allowing a reduction in the intra-erythrocyte concentration of HbS, thus delaying its polymerization rate. These results partly explain the effects of flavonoids on HbS polymerization.
Our results also indicate that total flavonoids from J. secunda stabilize the membrane better than anthocyanins from the same plant with the median corpuscular fragility of 0.63; 0.728 and 0.732 for anthocyanins (Mpiana et al., 2010), flavonoids, and disodium cromoglycate used as positive control, respectively.
Inhibition of sickle hemoglobin (HbS polymerization)
The polymerization rates of HbS and its inhibition are presented in Table 5.
Table 5
Polymerization rates of HbS and its inhibition by crude flavonoids extracted from Justicia secunda and disodium cromoglycate (n = 3 technical replicates).
Table 5 demonstrates that the polymerization of sickle hemoglobin (HbS) is partially inhibited in the presence of both disodium cromoglycate and crude flavonoid extracts of Justicia secunda, with comparable efficacy.
Mpiana et al. (2010) previously reported that under hypoxic conditions, anthocyanins from J. secunda effectively inhibit HbS polymerization. Specifically, anthocyanin extracts led to an increase in absorbance at 540 nm from 0.470 to 0.506, representing an 8% increase. In this study, crude flavonoids extracts resulted in an absorbance increase from 0.307 to 0.590, corresponding to a 37% increase. These findings confirm the significant anti-sickling activity of the total flavonoids, highlighting their potential in managing sickle cell disease.
Modulation of methemoglobin formation
Table 6 and Figure 8 show the evolution of methemoglobin as a function of time.
Table 6
Modulation of methemoglobin formation in the presence of flavonoid extracted from Justicia secunda and disodium cromoglycate (mean ± SD; n = 3 technical replicates).
Figure 8
Changes in hemoglobin andmethemoglobin levels over time. Bars represent the mean ± SD for N=3.
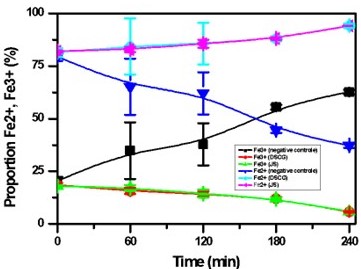
The results presented in Table 6 and Figure 8 reveal that under hypoxic conditions, the Fe²⁺/Fe³⁺ ratio is low in the negative control, confirming decreased oxygen affinity of HbS, leading to its polymerization and red blood cell sickling. However, in SS blood samples treated with disodium cromoglycate and Justicia secunda flavonoid extracts, the Fe²⁺/Fe³⁺ ratio is elevated, indicating that these flavonoids may prevent erythrocyte sickling by reducing in vitro oxidation of hemoglobin. This suggests significant antioxidant activity, a well-known property of polyphenols, including flavonoids (Biapa et al., 2019; Nanfack et al., 2013). Quercetin and kaempferol, key flavonoids, protect RBCs against hemoglobin oxidation and lipid peroxidation, thus reducing oxidative damage and inflammation in sickle cell disease (Mishra et al., 2022). These findings align with existing literature that highlights the role of traditional medicine plants in treating sickle cell anemia, showing benefits such as reduced reactive oxygen species (Alabi, Adegboyega, Olawoyin, & Babatunde, 2022; Gonçalves et al., 2024; Hassanpour & Doroudi, 2023; Mishra et al., 2022), stabilized erythrocyte membranes (Delesderrier et al., 2020), and decreased polymerization and hemolysis (Ohiagu, Chikezie, & Chikezie, 2021). Despite their biological activities, flavonoids' absorption and metabolism in the body are not fully understood, as they may undergo significant modifications that impact their efficacy (Hollman, 2004; Sak, 2023; Thilakarathna & Rupasinghe, 2013). This study underscores the substantial therapeutic potential of Justicia secunda from the DRC for managing sickle cell disease, validating its antisickling properties and highlighting the importance of traditional medicine in addressing this prevalent condition.
Conclusion
The high cost and limited availability of conventional treatments, coupled with difficulties in accessing these treatments, have prompted the exploration of indigenous knowledge for discovering plant-derived molecules capable of inhibiting HbS polymerization, which is responsible for sickle cell crises. Justicia secunda, traditionally used in various Congolese medicinal systems, has been the focus of this investigation. Phytochemical analysis by LC-MS/MS has revealed 12 flavonoid O-glycosides in the leaves of J. secunda, including one flavonol (kaempferol) and two flavones (luteolin and apigenin). The crude flavonoid extract exhibited significant inhibition of sickling, polymerization, osmotic fragility, and antioxidant activities. The results of the present study support the traditional use of J. secunda by indigenous populations in the DRC. However, attributing the anti-sickling activity solely to flavonoids is challenging, as previous studies have indicated that anthocyanins are also responsible, suggesting a possible synergistic effect of different compounds. This research aligns with the WHO's 2014-2023 strategy, which advocates for the modernization of complementary and alternative medicine. To ensure safety, particularly for pregnant women, further studies on teratogenicity and prenatal toxicity are warranted, given the traditional use of J. secunda for its abortifacient properties. Although the research indicates that flavonoids from J. secunda may mitigate sickle cell crises, understanding their precise mechanism of action remains essential. Future molecular dynamics simulations could provide insights into how these compounds interact with proteins involved in sickle cell pathophysiology.
Author contributions
Gbolo B.Z. designed and carried out the study, conducted the experiments, analysed the data and wrote the manuscript. Semay I. and Gerbaux P. carried out the LC-ESI/MS/MS analysis. Mpiana P.T., Duez P. helped to design the study and supervised it. Ciala N.B. and Ngbolua K.N. carried out biological experiments. All authors read and approved the final manuscript.
Funding
This project was carried out thanks to the funding of the Academy of Research and Higher Education (ARES) in its program "Institutional Support (IA) 2018-2020". Grant number: COOP-CONV-18-004, AI ARES UNIKIN, of which Mr. Gbolo was the main beneficiary and part of this study was granted by Wallonie-Bruxelles Internationale (WBI) through the project TradiQual.