Introduction
Mycoses are a serious danger to human health; they could impact different body parts. The severity of these infections differs significantly depending on the variety of fungi, the individual's immune system, and the location of the infection (Manuela Oliveira et al., 2023). To manage these infections, researchers are exploring natural alternatives to chemical antifungal drugs, to minimize their negative effects on health and improve people's quality of life (Byrne et al., 2022). Lactic acid bacteria (LAB) are gaining increasing interest because of their long history of secure application and antimicrobial qualities. They inhibit fungal growth by producing various compounds (Liu et al., 2022a). Additionally, some strains of LAB have shown the ability to eliminate mycotoxins by producing degrading enzymes or binding them to bacterial cell wall components (Sadiq et al., 2019). Furthermore, LAB could exert a probiotic effect, offering a range of health benefits. They have demonstrated anti-obesity effects through the production of exopolysaccharides, hypocholesterolemic effects, and the production of hypotensive peptides for cardiovascular disease prevention (Al-Sheraji et al., 2012; Lim et al., 2017; Wu et al., 2017). Moreover, LAB exhibits anti-carcinogenic properties, helps in the prevention of colon and breast cancers, shows casing antioxidant activity, and inhibits pathogen proliferation (De Moreno De LeBlanc and Perdigón, 2010; Thibault et al., 2004; Zhang et al., 2017). In addition to all these benefits of LAB, this study will focus on one of the species renowned for its antibacterial effects, maintaining intestinal health and improving the host's immune system: Lactiplantibacillus plantarum.(Behera et al., 2018; Karczewski et al., 2010; Kim et al., 2021)
Given the growing need to combat fungal infections and reduce dependence on synthetic antifungal drugs, current work aims to identify and isolate active LAB strains and characterize their antifungal compounds using a range of treatments, FTIR, UV and HPLC, then determine the effect of these compounds on the morphology of Candida albicans using scanning electron microscopy, to offer a biological alternative for the treatment of mycoses.
Materials and methods
Isolation of lactic acid bacteria (LAB)
A range of 35 biotopes including seeds, fruits, fermented vegetables, meats and milk of cow, sheep, goat, and camel have been chosen for the isolation, due to their availability in the local market and their increased potential content of lactic acid bacteria (Whitman, 2009). Samples were introduced to 90 mL of MRS broth (De Man et al., 1960) (Biokar, Beauvais, France), and after a 48–72h incubation period at 30°C, they were successively dissolved (0.1 w/v) in peptone solution, then 100 microliters of each suspension were streaked onto selective MRS agar (Biokar, Beauvais, France) with 1% calcium carbonate added as a supplement to detect, after incubation, colonies of LAB and to rule out non-LAB contaminants during the enrichment and isolation process. Colonies surrounded by a clear zone and showing different phenotypes were selected for purification through recurrent streaks on MRS agar plates, then a microscopical examination preceded by Gram coloration and catalase test were carried out for colony purity assessment. Only isolates that were catalase-negative and Gram-positive were selected, and they were placed in MRS broth containing 20% glycerol at a temperature of -80°C for prolonged storage.
Fungal strains
Six fungal species tested in this study were selected because of their potential pathogenicity to cause human mycoses (Kamtalwar et al., 2023; Ma et al., 2024; M. Oliveira et al., 2023; Sun et al., 2023). These are Aspergillus fumigatus175134378- 02 (A.fumigatus) from the mycology laboratory at Angers University Hospital (France); Fusarium oxysporum IHEM 9571 (F.oxysporum) collected from the Institute of Hygiene and Epidemiology in Belgium as well as the mycotheque of the Catholic University of Louvain; Penicillium brasilianum (P.brasilianum) from the University Moulay Ismail (Morocco), Aspergillus niger (M100) (A.Niger) from the national center of scientific and technical research (CNRST), (Morocco); Candida albicans (C.albicans) and Candida non-albicans (C.N.albicans) from Moulay Ismail military Hospital (Morocco).
Suspensions of fungal spores and yeast cells
fungi strains were cultivated on Potato dextrose agar (PDA, Biokar) and maintained at optimum temperature for each species in question (i.e., 30 °C for Aspergillus fumigates and A.niger, 25 °C for Penicillium brasilianum and fusarium oxysporum) for 7 days (until sporulation); While, The yeast were grown for 24 hours at 30 °C in malt extract broth (Biokar). After incubation, the fungal spores were collected by washing the agar medium's and the concentration of the spores and yeasts was adjusted to 106cells/mL.
In vitro antifungal effect
Tests were conducted on the LAB strains' inhibitory activity against fungus using the overlay method described by Magnusson and Schnürer, (2001). Firstly, LAB isolates were assessed against C.albicans, as an indicator species because of its pathogenicity. So, LABs were spread on MRS agar medium in 2 cm intersected lines, and maintained anaerobically for 48 hours at 30 °C; then, 10mL of malt extract soft agar containing 1 milliliter of yeast or fungus inoculum were transferred to the agar layers. Following 24 hours of incubation in aerobic conditions at 30 °C, the presence or absence of clear inhibition zones surrounding the LAB lines was measured and noted (Magnusson et al., 2003) as follows:
(-) : lack of inhibition (⌀<1mm);
(+) : inhibition of fungal growth on 0.1- 3% of the Petri dish surface / LAB streak (1mm≤ ⌀<2.6mm);
(++) : no fungal growth on 3- 8% of the Petri dish surface / LAB streak (2.6mm≤ ⌀<7mm);
(+++) : no LAB fungal growth on> 8% of the Petri dish surface / LAB streak (⌀>7mm).
The non-inoculated MRS with LAB, served as a blank control, and each test above was performed three times.
Antifungal activity spectrum
Bacterial strains that demonstrated effect towards C.albicans were used to evaluate their effects to inhibit the other five fungis. The method used is similar to that described above. Each inhibition test was performed in triplicate.
Evaluation of antifungal activity in cell free supernatant (CFS)
Preparation of CFS
The method used is that of Magnusson et al., (2003) briefly modified; a LAB strain colony was first added to 10 milliliters of MRS broth and kept at 30°C for 18 hours. Then 1mL of this culture has been added to 100 mL of MRS and incubated for 48 hours at 30°C; the resulting culture was centrifuged at 6000g for 15 minutes, and then passed through the filter (0.22 μM, Millipore). The sterile CFS were then freeze-dried and re-suspended in sterile distilled water to obtain solutions with concentrations from 5 to 25-fold concentrated CFS relative to the initial volume. The cell free supernatant solutions were stored at 4°C until their utilization (Arrioja-Bretón et al., 2020).
Antifungal effect against C. albicans by well diffusion approach
To evaluate the antifungal activity of CFS, we used the agar well diffusion technique suggested by Magnusson and Schnürer, (2001); Thus, Petri dishes containing PDA were submerged with 1mL of C.albicans (106 cells /mL). Following drying, 100 μL of LAB-CFS was added to 6 mm diameter wells made in the agar utilizing a sterile cork-borer. Following an incubation period of 24 hours at 30°C, the diameter (mm) of the inhibitory areas surrounding the wells was recorded. Each manipulation was done in triplicate.
Characterization of LAB strains with high anti-fungal activity and broad spectrum
Lactic acid bacteria strains that showed inhibitory activity and a broad antifungal spectrum were taken up for identification using the sequencing of the 16S ribosomal gene (16S rDNA). Thus, the chosen strains were grown on MRS agar (Biokar) for 72h at 30°C, and the bacterial DNA extraction was carried out utilizing the procedures required by Moroccan standard NMISO 20837-2008. The results for the concentration and quality of DNA extracted from strains are obtained by utilizing a Nanodrop 8000 UV-Vis spectrophotometer. 16S rDNA of the selected isolates was amplified by PCR using Green-Taq DNA polymerase (canvax, Cordoba, Spain). Universal primers pA (5’-AGAGTTTGATCCTGGCTCAG-3’) and pH (5’- AAGGAGGTGATCCAGCCGCA-3’) were used to amplify the 1500 bp fragment of 16SrDNA. 20μL of each sample contains a mix of 10μL 2x Green- Taq mix, 1 μL of forward primer (15 μM), 1 μL of reverse primer (15 μM), 25-500ng of DNA template, and autoclaved Milli-Q water. A first denaturation at 94°C for 5 minutes was followed by 35 cycles of denaturation at 94°C for 30 seconds, annealing at 56°C for 30 seconds, extension at 72°C for 30 seconds, and a final extension at 72°C for 5 minutes, were the steps of the PCR reactions. PCR products were then photographed after electrophoresis in a 1% agarose gel. Following that, the Exo SAP-ITM PCR Product Cleanup Reagent kit was used to purify the PCR products, regarding the procedures specified by the manufacturer, and sequencing has been carried out adopting the Sanger approach as modified by the Big DyeTM Terminator v3.1 Cycle sequencing kit. Finally, Seq Studio Genetic Analyzer (Applied Biosystem, Foster City, CA, USA) was utilized for automatic analysis of sequence reactions.
Phylogenetic affiliation of strains is obtained by comparing sequences with GenBank database using the BLAST for strains assignment. Sequences were then compared to our study’s strains and recorded in the GenBank database.
Physicochemical characterization
Physicochemical characterization was performed by studying the growth of LAB incubated for a period of 24 to 72 hours in MRS broth at varied pH (from pH3 to pH11), NaCl concentrations (from 0% to 9%), and temperatures (from 15 to 45°C). After incubation, the optical density of each culture broth was measured (Ani et al., n.d.). The measurement of percentage growth was taken using the following Eq.1 (Bulgasem, B. Y. et al., 2017):
With At is the OD560 measured respectively after 24, 48 and 72 hours while A0 is the OD560 measured in the beginning of the experiment.
Characterization of antifungal compounds
The nature and properties of antifungal compounds are determined through examining them in a CFS (Shehata et al., 2019). The stability of compounds under various conditions is evaluated using neutral pH and heat treatment, while the possible protein nature of the compounds can be identified through the application of proteolytic enzymes. HPLC makes it possible to precisely separate and quantify active compounds, while FTIR and UV analysis offer information on functional groups and chemical structures. Defining their mechanism of antifungal action and their potential in biotechnological or medical domains requires an in-depth description, which these combined methodologies provide.
Enzymatic treatment of antifungal compounds
The chemical properties of the antifungal substances were examined by applying the approach of (Ahmad Rather et al., 2013), with slight modifications. Cell-free supernatant (CFS) from the three strains were twenty-fold concentrated and submitted to various procedures, such as neutralizing pH7 and exposure to catalase, pepsin, and proteinase K for 3h at 37° C. The enzyme concentration was 1 mg/mL in CFS and the pH values were modified to optimal pH for each enzyme. The treated CFSs were tested against C. albicans using the well diffusion technique. Every CFS's pH was changed to its initial value before analysis. The control used was the 20-fold concentrated CFS and each experience was carried out in triplicate.
Characterization of compounds functional groups by FTIR
An FTIR (Fourier Transform InfraRed spectroscopy) was utilized for the identification of the functional groups and the elucidation of chemical structures contained in the three CFSs of the active LABs by the recommended operational protocol (Palaniappan et al., 2015). This groups were identified by scanning the sample from 500 to 4000 cm-1 with a 4 cm-1 KBr pellet approach (Al-Ansari et al., 2023; Palaniappan et al., 2015).
Characterization of chemical compounds by UV-visible
Using a UV-2600 ultraviolet-visible (UV-vis) spectrophotometer, We determined the samples' UV-Vis absorption bands at 25°C with phosphate buffer solution (Chen et al., 2019).
Organic acid quantification using HPLC-UV
High-performance liquid chromatography with ultraviolet (HPLC-UV) detecting was used to determine organic acids. For the chromatographic separations, a PerkinElmer Altus™ HPLC System was used with detection in 210nm. Carrez I and II solutions were used for precipitating the proteins contained in the CFS, and the mixture was then centrifuged for 10 minutes at 4000 g. The supernatants were diluted with HPLC-grade water, followed by passing through a 0.45-μm polyamide filter before being examined using a PerkinElmer AltusTM HPLC System. Every diluent, reagent, and solvent used was of HPLC standard and was filtered. A column C18 5-μm (250 x 4.6-mm) with 1.5 mL/min flow at 30°C was utilized for separation. For the first mobile phase, 10-mM KH2PO4, pH 2.4 with sulfuric acid was applied, and for the second mobile phase, Acetonitrile (ACN). At least 4 levels of standard solutions including the following acids: acetic acid, propionic acid, formic acid, and maleic acid have been used for calibrating in the range of 0.5– 25 mg/L. The employed standard solution was serially diluted to give six calibration levels. Each 20 μL of the standard level was injected in duplicate.
Anti-candida activity of CFS
Effect on C.albicans growth
Cell-Free Supernatant (CFS), of some LAB, has fungicidal and fungistatic properties that prevent the growth of Candida species and alter their morphology. Fungal infections are controlled by the fungistatic effect, which slows down the growth of fungal cells, while the fungicidal effect destroys fungal cells (Bianchini, 2015).
The three LAB strains with the highest antifungal activity were examined for their activity against C.albicans as an indicator. 1 ml of each CFS was inoculated in 50 μL of C.albicans suspension (105cells/mL) and incubated for 05 to 30 minutes, and from 1h to 24 hours at 30°C; after that, ten μL of that mixture was poured into a PDA plate to test the C.albicans survival. A control is carried out under the same conditions using sterile MRS instead of CFS.
Effect on C.albicans morphology
Frequently, the mechanisms of LAB action against fungus manifest themselves in structural modifications, such as deformation or damage to cell walls (Balkis et al., 2002). The advantage of SEM is that it enables precise visualization of cellular effects, providing a better understanding of antimicrobial mechanisms of action (Relucenti et al., 2021).
Scanning electron microscopy (SEM) was used to explore the effect of LAB-CFS on C.albicans morphology, with the approach of (Ahmad Rather et al., 2013) slightly adapted. At first, 1 ml of C.albicans inoculum (105 cells/mL) was sprayed on three dishes containing PDA medium. Each plate had two 1cm-diameter filter papers positioned on either side of its center. Next, 100µL of five-fold concentrated CFS from each of the 2GP, 4FB, and 10SB bacterial strains were added to a paper disc of one of the three plates. The three filter papers were taken out carefully and immersed for 4 hours at 4°C in a 2.5% glutaraldehyde solution. Following six repetitions of a 20-minute wash with 0.2 mol/L sodium phosphate, they were then incubated for an additional night at 4°C in a 1% osmic acid solution. The three samples were dried with carbon dioxide after being submerged in isoamyl acetate 2 times for twenty minutes each. Finally, the samples were viewed under a Nova Nano SEM 650 scanning electron microscope after being placed on aluminum supports and coated with gold. For comparison, control plates with filter paper devoid of CFS were also treated.
Results
LAB isolation and antifungal activity spectrum
300 LABs were isolated from 35 different biotopes from January 2020 to March 2021. They are Gram+, catalase-, and surrounded by a clear area in MRS Agar supplemented with CaCO3. When tested towards the indicator fungus C.albicans, 32 strains (10.42%) showed antifungal activity, 14 (4.56%) of them demonstrated a wide antifungal spectrum. However, three isolates of these LABs stand out from this group, they present inhibitory activity against all the fungi tested and a large inhibitory activity against C. albicans; they are designated 2GP, 10SB, and 4FB and were isolated respectively from bee pollen, white sorghum, and prickly pears. Figure 1 illustrates the antifungal activity of the isolates 2GP, 10SB, and 4FB, and Figure 2 provides an overview of the LAB inhibitory spectrum findings and their ability to suppress fungal growth.
Figure 1
Antifungal activity of LABs isolates 2GP (a), 4FB (b), and 10SB (c) against Candida albicans.
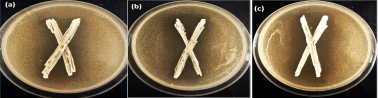
Figure 2
Inhibition zones map of thirty-two LABs against six fungal strains. (-): Lack of inhibition; (+): lack of fungal growth on 0.1–3% of Petri dish zone around LAB streak; (++):lack of fungal growth on 3–8% of Petri dish zone around LAB streak; (+++):lack of fungal growth on > 8% of Petri dish zone around LAB streak. Data are the mean ± standard deviation of at least 03 independent experiments.
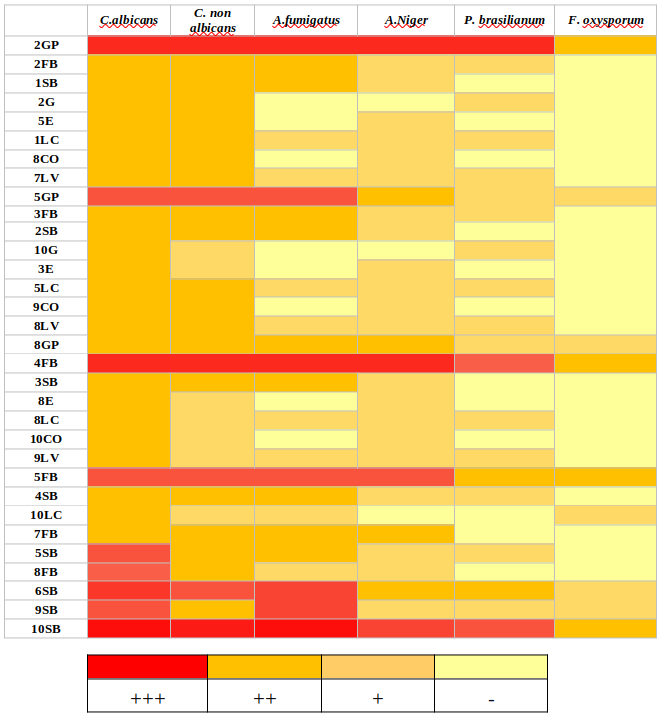
Morphological and molecular identification of LABs with high antifungal activity
The three LAB isolates 2GP, 10SB, and 4FB exhibit bacillary morphology; their partial studying of the 16S rDNA gene classifies them within the genus Lactobacillus and the species Lactobacillus plantarum. Observedly, it must be mentioned that the name Lactobacillus plantarum was just modified to Lactiplantibacillus plantarum (Zheng et al., 2020). Table 1 shows 16S rDNA sequencing results of the three strains with high antifungal activity.
Table 1
Partial sequencing 16S rDNA gene results for strains with high antifungal activity
16S rDNA | Similarity (%) | GenBank accession number | |
---|---|---|---|
2GP | Lactiplantibacillus plantarum | 99.19 | PP901278 |
4FB | Lactiplantibacillus plantarum | 99.27 | PP901503 |
10SB | Lactiplantibacillus plantarum | 98.87 | PP901856 |
To determine the degree of relatedness between the three LABs strains, we carried out a Neighbor-joining method phylogenetic study of 16S rRNA gene sequences; the results obtained are shown in Figure 3.
Physico-chemical characterization
Physico-chemical characterization demonstrated that the three LABs strains grows in various degrees of pH from 3 to 11 with optimal growth in pH5 and a temperature range from 20°C and 45°C. Regarding the hyaline medium, bacterial strains showed growth in the presence of a NaCl concentration ranging from 0 to 8% with optimal growth of 5% for 10SB and 4FB and 4% for 2GP.
Antifungal activity of the CFSs
Using the agar well diffusion method, the concentrated CFS of the three LABs 2GP, 4FB, and 10SB were examined for antifungal activity against C.albicans. The obtained results showed the presence of a halo devoid of fungal growth around each diffusion well (Figure 4); this suggests the inhibitory action of molecules contained in the CFS.
Identification of antifungal compounds in CFS
pH neutralization and enzymatic treatment of CFS
The pH Neutralization and enzymatic treatment of CFS showed, in each case, a reduction in antifungal activity, suggesting that CFS contains compounds of acidic and protein nature as well as the presence of H2O2 (Figure 5).
Functional groups characterization using FTIR
The infrared spectra of bacterial strains 2 GP (black), 10SB (blue), and 4FB (red) are presented in Figure 6. The main peaks indicate the functional groups of CFS chemical compounds. A peak at 3748.90 cm-1 may be related to succinic acid, benzene propanoic acid, or the carboxylic acid of L-lactic acid (Anu and Vijay, 2016), and another peak, located at around 3322.27 cm-1, might be the O-H stretching vibration found in 2-hydroxy-4-methyl pentanoic acid (Maliszewska et al., 2024) and can be originated from protein and carbohydrates present in CFS of Lp.plantarum (Mohd Yusof et al., 2020). Unsaturated C-H bending vibrations in pentanoic acid, 2-hydroxy-4-methyl, benzene propanoic acid, hexadecanoic acid, succinic acid, methyl stearate, and L-lactic acid may be responsible for another peak at approximately 2115.53 cm-1 (Gao et al., 2021) and can correspond to the presence of free carboxylic groups in CFS (Abo Saif and Sakr, 2020). The aromatic ring's C=C stretching vibration in propanoic benzene acid may be represented by peaks at 1633.37 cm-1 and 1573.46 cm-1 (Yin et al., 2023). Benzene propanoic acid, succinic acid, pentanoic acid, hexadecanoic acid, methyl stearate, 2-hydroxy-4-methyl and L-lactic acid may all have C-H and C-O stretching vibrations, which could explain the peaks between 1456.48 cm-1 and 1313.83 cm-1 (Gao et al., 2021). The -CHOH stretching vibrations of pentanoic acid carbohydrates, 2-hydroxy-4-methyl, or L-lactic acid may be represented by the peaks between 1263.90 cm-1 and 1041.36 cm-1 (Maliszewska et al., 2024). Last but not least, the peak around925.8 cm-1 may represent the stretching vibration of branched hydrocarbons or the decomposied aliphatic hydrocarbons (Yin et al., 2023).
Functional groups characterization using UV
The study of the CFS UV spectra of the three strains (2GP, 4FB, 10SB) defined the existence of a specific band at 205 nm in line with the n-π* transitions of carboxylic acid in all three samples (Figure 7). On the other hand, sample 2GP shows two other bands appearing at 232 and 270 nm, representing the n-π* transitions of C=O bonds and the π-π* transitions of aromatic C=C bonds, respectively. Three characteristic bands are observed in the UV-Vis spectrum of 4FB at 232, 247, and 290 nm, representing respectively to the π-π* transitions of the aromatic C=C bonds, the π-π * transitions of the aromatic ring, and the n-π* transitions of the C=O bonds. The results of the UV-visible spectrum analysis of sample 10SB revealed the presence of a band around 250 nm corresponding to the π -π * transitions of the aromatic ring, as well as another one around 295 nm corresponding to the n-π * transitions of the C=O bonds.
Identification of organic acids by HPLC-UV
CFS analysis, using the HPLC-UV method, showed that the CFS contained seven organic acids of Lp. plantarum 2GP, 4FB, and 10SB; however, it is lactic and acetic acids that are dominant in the three lactic acid bacteria CFS (Figure 8). Oxalic acid and succinic acid are defined according to (Ergönül and Nergiz, 2010). Table 2 shows retention time, concentration, and peak area for the Five organic acids.
Figure 8
HPLC chromatograms of organic acids in the CFS of the three LABs 2GP, 4FB, and 10SB. 1. Formic acid, 2. Oxalic acid, 3. Lactic acid, 4. Acetic acid, 5. Maleic acid, 6. Succinic acid, and 7. Propionic acid
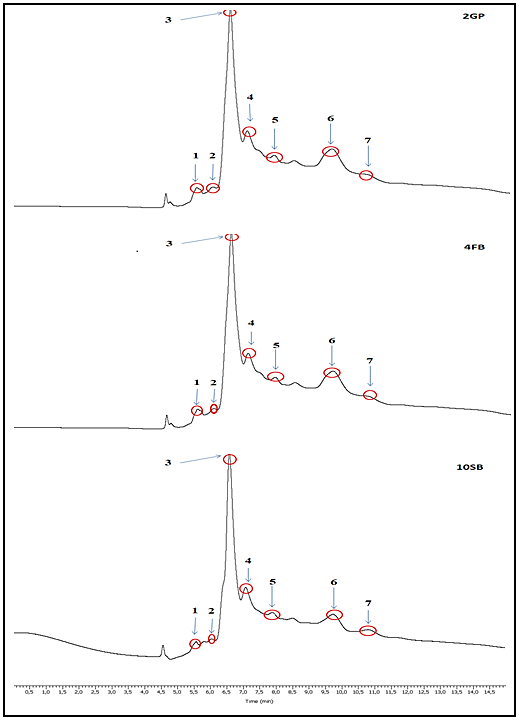
Table 2
Retention time, concentration, and peak area of five organic acids from Lp. plantarum strains 2GP, 4FB and 10SB.
Fungicidal and fungistatic effect of CFS
Effect on fungal growth
Fungicidal action against C. albicans was demonstrated by the cell-free supernatant of the three Lp.plantarum strains. Lack of yeast growth was noticed after 30 minutes of interaction concerning the three LABs CFS and the C. albicans strain. It should be noted that this time is reduced to only 10 min for strain 10SB, indicating the strong inhibitory activity of its CFS. Figure 9 shows the fungicidal or fungistatic effect of the three LABs CFS against C. albicans after different contact times.
Effect on C. albicans morphology and structure
SEM analysis of C.albicans treated with Lp.plantarum 4FB CFS showed notable morphological and structural modifications resulting in yeast cell deformations (D), accompanied by a decrease in size (C); we also note the absence of buds and both filamentation and extracellular film, resulting in dispersed deformed yeast cells (C). However, untreated C. albicans cells have no morphological alterations and appear as mature yeasts (B), they exhibit extracellular layer and bud formation as a sign of multiplication activity. At a resolution of x5000, the cells are easily visible (A). It appears that SEM analysis is in concordance with previously reported fungicidal results (Figure 10).
Figure 10
Scanning electron photomicrographs showing the effect of Lp. plantarum 4FB CFS on C.albicans (1) extracellular matrix, (2) Bud scar, (3) Bud, (4) Birth scar, and (5) cell alterations. (A) Untreated filter × 5000; (B) Untreated filter × 7000; (C) Treated filter × 7000; (D) treated filter × 40,000.
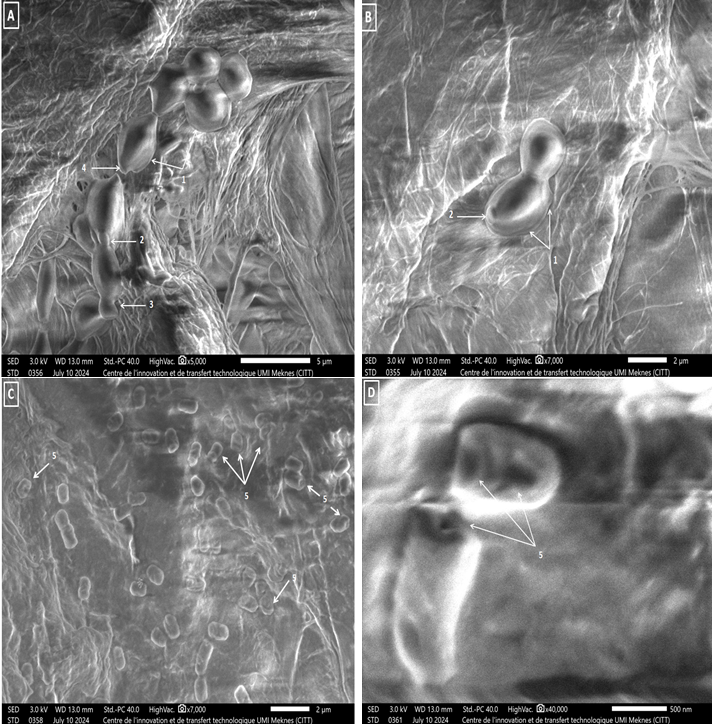
Discussion
Fungi have a close evolutionary relationship with human hosts, finding new antifungal targets is more difficult than it is for bacteria (Kanafani and Perfect, 2008). Generally, an antifungal needs many years to be approved for clinical use and efforts to decrease toxicity, boost bioavailability, enlarge the antifungal spectrum, and find solutions to resistance should all contribute to the effectiveness of currently available antifungals (Van Daele et al., 2019). Because of this, alternative treatments for fungal infections, such as the use of oils, phytochemicals, and peptides have been tested, but unfortunately, more studies are necessary due to the bio-tolerance and toxicity of these compounds (Divyashree et al., 2023).To rectify this situation, an alternative strategy involving bio-control agents such as LAB has been predicted for treating opportunistic human fungal infections (Matsubara et al., 2016).
300 LAB were isolated from 35 biotopes and evaluated for their antifungal activity against various fungus involved in human mycoses such as C.albicans which was chosen primarily as the target mold for the initial screening. Thus, the screening results allowed us the select three Lactiplantibacillus plantarum strains with high antifungal activity; they are designated in this work 2GP isolated from bee pollen, 4FB from Opuntiaficus-indica fruits (prickly pear) and 10SB from white sorghum. It should be noted that most Lp.plantarum strains are ubiquitous, occupying most biotopes at variable frequencies and known for their antifungal activity but to varying degrees (Li et al., 2023; Russo et al., 2017; Zha et al., 2024). however, biotopes such as bee pollen, white sorghum, and Opuntiaficus-indica fruits have rarely been the subject of isolation of such bacteria, moreover, strains of this species with notable antifungal activity have not been, so far, reported by previous scientific works (Barba et al., 2022; Di Cagno et al., 2016; Verón et al., 2017; (Ispirli and Dertli, 2021). Furthermore, the fungal inoculum used (106 spores/mL) for the antifungal tests, is well above the level expected in the natural environment. By measuring the diameter of the zone of inhibition on agar and the variety of fungi that our strains can inhibit, we have demonstrated that our lactic acid bacteria strains, 2GP, 4FB, and 10SB, have effective antifungal activity against six fungi that are pathogenic to humans. Our results lines up with those of other researchers who have isolated and examined lactic acid bacteria that have antifungal properties (Riolo et al., 2023; Simões et al., 2023; Souza et al., 2023). Indicating that the strains isolated in this work have great antifungal potential. Physicochemical characterization shows that the three isolates selected were able to grow at pH values between 3 and 11, and MRS broth contains between 0% - 8% NaCl concentrations. In addition, they can grow at temperatures between 20°C and 45°C; such characteristics make these LABs excellent biological tools resistant to possible stressful environmental conditions.
The CFS of the three Lp.plantarum strains was subjected to a series of enzymatic treatments to determine which substances are responsible for the antifungal activity; thus, pepsin and proteinase K treatments indicate the potential contribution of proteinaceous compounds in such activity. Indeed, after CFS treatment with these enzymes, the antifungal activity was reduced; these findings are in accordance with the results from previous studies (Abouloifa et al., 2022; Hernández-Figueroa et al., 2024). Wang et al., 2024, mentioned the non-involvement of proteinaceous compounds in antifungal activity. Similarly, CFS treatment with catalase and its neutralization to pH 7 showed a decrease in antifungal activity, suggesting the involvement of both hydrogen peroxide and organic acids in this activity; the involvement of these compounds in antifungal activity has also been reported by (Sedaghat et al., 2016). It should be noted that organic acids inhibit fungal activity by penetrating cell membranes and accumulating in the cytoplasm, this leads to the inability to produce biological energy, which inhibits fungal growth (Piper et al., 2001).
Organic acids, fatty acids, and peptide compounds are examples of active metabolites that can be produced by LABs. The final products of fermentation include lactic, acetic, propionic, and other organic acids, which create an acidic condition preventing the formation of pathogenic microorganisms (Chen et al., 2021). FTIR, UV-visible, and HPLC-UV were then used for antifungal compound identification. Firstly, a few research have used FTIR analysis to investigate CFS; FTIR analysis showed an identical spectrum for the three Lp.plantarum strains; this result shows that even though the strains come from different biotopes, this does not affect the nature of the chemical compounds contained in their CFS, which may be organic acids, peptidic compounds, and/or carbohydrates; the same observation was made by the authors (Mohd Yusof et al., 2020). However, Madhu and Prapulla (2014) report that the chemical compounds secreted by Lp.plantarum strains may not be similar (Li et al., 2023; Muhialdin et al., 2011; Nayyeri et al., 2017). CFS analysis, using the HPLC-UC method, showed seven organic acids synthesis by Lp. plantarum 2GP, 4FB, and 10SB, these include lactic, acetic, propionic, succinic, formic, oxalic, and maleic acids however, it is lactic acid followed by acetic acids which are synthesized in relatively large quantities; an identical result was reported by (Nasrollahzadeh et al., 2022); however, some research reports the effectiveness of acetic acid over lactic acid in antifungal activity (Liu et al., 2022b; Pangprasit et al., 2020). The Other organic acids also exhibit antifungal activity, in fact, according to (Lastauskienė et al., 2014), formic acid induces C.albicans cell death, and the antifungal activity of oxalic acid is demonstrated by Yamaji et al., (2005). Maleic acid can inhibit the germination of fungal mycelium, while succinic acid has been shown to have an inhibitory effect on both bacteria and fungi. Propionic acid also has antifungal properties; it can cause oxidative stress and mitochondrial dysfunction in fungal cells. (Chen et al., 2011; Kamzolova et al., 2014; Yun and Lee, 2016). Although the UV-vis spectra of the three Lp.plantarum strains CFS show an almost similar appearance, the analysis shows the presence of a few differences. The n-π* transitions of the carboxylic acid-like formic, acetic, and propionic acids were represented by a band at 205 nm. Singleton et al., found the absorption to be a maximum at 215 nm, which was discovered in the CFS of the three strains, and they also show the n-π* transitions of the C = O bonds, but at different wavelengths. Only strains 4FB and 10SB show the π-π* transitions of the aromatic ring present in aromatic compounds like volatile phenols (Goswami et al., 2018). The fungicidal effect of Lp.plantarum 2GP and 4FB CFS on C. albicans was observed in this study after 30 minutes of incubation; however, for Lp.plantarum 10SB strain there was no fungal growth after just 10 min of contact; this inhibition is due to a wide variety of antimicrobial metabolites produced by LABs; they act by causing membrane depolarization, inhibition of cell wall formation, cell wall lysis, and proteins and nucleic acids destroy. They also cause oxidative stress and lower ATP levels inside cells. Similarly, LABs create antifungal compounds that cause deformation to the cell surface, damage to the fungal cell's hyphae, and cause cytoplasmic material leakage. (Kaveh et al., 2023; Mani‐López et al., 2022; Zapaśnik et al., 2022).Consequently, plantaricin E/F, which can be produced by Lp.plantarum, may be responsible for the fungicide effects seen in the present study. Furthermore, Lp.plantarum has been supposed to contain terpenes, aromatic polyketides, and cyclic lactone autoinducers (Bae et al., 2024). The ability to alter filamentous and yeast morphologies impacts C.albicans pathogenesis, and this transition is essential for virulence (MacAlpine et al., 2021). Lactiplantibacillus sp and other microbiota members are interacting with C. albicans to control virulence (Alonso-Roman et al., 2022; Oliveira et al., 2016).
The analysis of scanning electron microscopy confirmed the previously reported fungicidal results. Our study showed an inhibitory effect of Lp.plantarum 4FB CFS on Candida growth.
This CFS caused a deformation and destruction of the C.albicans membrane. According to (Dong et al., 2024), the CFS of Lp.plantarum can destroy the cell wall and membrane, lead to mitochondrial damage and inhibit C. albicans; The fungicidal effect of CFSs is first due to the content of organic acids causing deformations and the size reduction in hyphae of the mold, and causing lesions on the mycelium (Mani‐López et al., 2022). This result supports those of other research with different strains and species of Lactiplantibacillus (Matsuda et al., 2018; Poon and Hui, 2023). We also note the absence of filamentation, these findings follow previous research that showed Lactiplantibacillus cells and CFS inhibited C. albicans filamentation (Ribeiro et al., 2017; Rossoni et al., 2018). It is known that C. albicans exhibits morphological differentiation in response to environmental pH. Acidic environments are ideal for yeast growth, while neutral and alkaline environments encourage hyphal growth (Davis, 2003). The influence of a slightly reduced pH on the filamentation inhibition, seen in our study, could not be ignored. The researchers additionally showed that the level of filamentation inhibition induced by Lactiplantibacillus CFS could not be achieved by acidic pH alone.
Conclusion
This study is centered on the isolation, identification, and characterization of lactic acid bacteria strains inhibiting the growth and development of fungi strains, particularly those responsible for human mycoses. Thus, three lactic acid bacteria strains identified as Lactiplantibacillus plantarum, designated 2GP, 4FB, and 10SB and isolated respectively from bee pollen, white sorghum, and Opuntia ficus-indica fruits have presented remarkable characteristics, not only of antifungal activity, but also of the antifungal spectrum; they present an efficacy in the inhibition and suppression of yeast responsible for human mycoses, in this case the species Candida albicans. The antifungal activity of these bacteria strains is due to compounds of various natures, secreted in the culture supernatant (CFS), these are protein compounds, hydrogen peroxide, and organic acids, which were approved by the diminution after treatments of the zone of inhibition from 19.333±1.528 mm to 4.333±1.155 mm for 2GP, from 20.667±1.155 mm to 5±1 mm for 4FB, and from 19.667±2.082 mm to 5.667±1.155 mm for 10SB. These compounds combined generate a fungicidal activity rather than fungistatic. The application of the Lp. plantarum strains, 2GP, 4FB, and 10SB, as a potential bio-alternative to chemical antifungal agents could represent a novel approach for treating human mycoses. Nevertheless, to completely understand the mechanisms, more in-depth molecular study needs to be conducted in the future.
Author Contributions
Ahmed Chbihi Kaddouri: Research concept and design, collection and/or assembly of data, data analysis and interpretation, writing the article, final approval of the article. Samia El Oirdi and Tarik Lakhlifi: Research concept and design, writing the article, critical revision of the article. Youssef Miyah and Mohammed Benjelloun: Data analysis and interpretation, writing the article, critical revision of the article, final approval of the article. Zakya M’hamdi: Collection and/or assembly of data, data analysis and interpretation. Abdelhaq Belhaj: Supervision, project administration, research concept and design, critical revision of the article, final approval of the article.