INTRODUCTION
According to the World Health Organization (WHO), more than 80 per cent of the African population relies on traditional medicine to solve primary health problems (Ngbolua et al., 2011; Ngbolua et al., 2011; WHO, 2002). This medicine is based on the use of medicinal plants, which have been used in various forms for thousands of years to treat common diseases. Their use represents important empirical knowledge that healers and practitioners are known only for (Bitsindou, 1996). Moreover, medicinal plants are versatile resources which can be used for food, nanotechnology, and agriculture, demonstrating their importance in various fields beyond traditional medicine (Gbatea et al., 2023; Kabengele et al., 2023; Mbula et al., 2023; Razafimahatratra et al., 2023).
The use of medicinal plant medicines is transmitted orally in traditional societies from generation to generation, and the risk of losing this knowledge is real, as biological resources are disappearing at alarming rates. The continent of Africa has a wealth of highly diverse medicinal plants, with more than 200,000 in Africa, many of which have medical properties (Sofowora, 1993). The development of these medicinal plants is of great concern to South African countries, as most of the population relies directly on traditional medicine and lacks adequate care, particularly in poor areas. Moreover, for certain diseases that modern medicine does not provide appropriate treatment, traditional recipes are an alternative (Masengo et al., 2022; Tshilanda et al., 2014). In fact, the antiviral activity of medicinal plants that treat respiratory diseases is scientific evidence (Mpiana et al., 2022; Mpiana et al., 2022; Ngbolua et al., 2020).
In tropical regions in general, and in the Democratic Republic of the Congo (DRC) in particular, influenza epidemics are recurrent. Influenza-related respiratory infection caused by influenza viruses is characterized by high genetic variability and the pathogenicity of this disease is due to the ability of the virus to induce disease in the infected host. This includes the ability of the virus to penetrate cells, replicate, and provoke an immune response, which can lead to symptoms such as fever, cough, fatigue, and muscle aches (Babakazo et al., 2019; Kavunga-Membo et al., 2015; Keilman, 2019; Meissner, 2001; Nkwembe-Ngabana, Ahuka-Mundeke, Kebela-Ilunga, Londa, & Muyembe-Tamfum, 2017). During the year, the seasonal outbreaks have been particularly frequent with a high mortality rate, leading to a growing concern over public health (Babakazo et al., 2019; Kavunga-Membo et al., 2015; Keilman, 2019). Moreover, the risk of pandemics associated with zoonotic influenza is a major concern for public health. In addition to hygiene measures, annual vaccinations remain the most effective protection. The disease mainly affects the lungs, nose and throat, particularly children, elderly, pregnant women, chronic diseases and those with weak immune systems (Keilman, 2019; Meissner, 2001; Nkwembe-Ngabana et al., 2017). In the DRC, the lack of flu vaccines has led to the use of medicinal plants by low-income people (Babakazo et al., 2019; Kavunga-Membo et al., 2015; Keilman, 2019; Meissner, 2001; Ngbolua et al., 2011; Ngbolua et al., 2011; Nkwembe-Ngabana et al., 2017). This study has been motivated by the high morbidity rates of influenza (because Coronavirus epidemic is a massive respiratory disease with influenza-like symptoms) in the central city of Kinshasa compared with the rural villages without significant health structures. The choice of Ibi village is justified by the fact that it is one of the few rural areas of Kinshasa experiencing different flu outbreaks in the city, thanks to easy and inexpensive herbal medicines. In particular, the environment is rich in plant diversity, including exotic and local species from forests and savannahs. Furthermore, the population living there is almost uniform and mainly composed of the Téké ethnic group. Due to their extensive indigenous knowledge of medicinal plants, the population has succeeded in managing various influenza and epidemics in the last three years in Kinshasa and has generated interest in the study. However, this disease can be confused with common illnesses such as malaria and typhoid fever, with which it shares the same symptoms (headache, fever, etc.). The use of medicinal plants to treat influenza could be beneficial for the poor population of Africa in general and the DRC in particular, which lacks basic health facilities such as respirators and essential medicines, especially at a time when the Coronavirus is wreaking havoc. The overall objective of this study is to identify traditionally used medicinal plants for influenza treatment in the village of IBI and its surroundings in the DRC. This will be achieved by combining an ethnobotanical approach with molecular validation through molecular docking and molecular dynamics simulation, aiming to assess their potential efficacy against influenza at the molecular level.
MATERIAL AND METHODS
Study area
Ibi Village is located in the Bateké Plateau, a large structural unit extending from Gabon to the DRC. Administratively, the Ibi village is located in the urban-rural district of Maluku, Kinshasa. It is 140 km east of Kinshasa City Centre, between 4°15 and 4°25 south latitudes, and between 16°4 and 16°12 east latitudes (Figure 1). The triangular Ibi village station covers over 20,000 hectares. The main highway (National Road 1) is 8 km away, and is easily accessible by car (Bisiaux, Peltier, & Muliele, 2009).
Material
In this study, all plants inventoried during survey constitute our biological material. These are plant parts (roots, stems, leaves) or whole plants used for the treatment of influenza-like illnesses.
Research methods
Ethnobotanical survey
This study used the snowball sampling technique. It consists of identifying the subject's competent informant, and after investigation, it indicates another competent informant in the same community. This process continued until the whole competent expert informant was investigated in the study (Mawunu et al., 2023; Ngbolua, 2020). The respondents were interviewed individually on the basis of a predetermined questionnaire form. The main collected data related to socio-demographic data (sex, age, level of education, marital status) and ethnocultural data (adjectives names, parts used, methods for making medical recipes, etc.).
Plant identification and classification
The majority of the plants were identified in the field, with the help of Professor Jean Lejoly, President of the non-governmental organisation GI Agro in Ibi-village. The identified plants were classified according to the APG IV system (IV, 2016).
Determining the consensus of informants and cultural importance
One of the techniques for assessing plant culture is the proportion of the agreement (or consensus) of informants, which takes into account the participants’ consensus and can therefore be used to evaluate the cultural importance of plants. In order to express the consensus or confidence index of respondents to medicinal plants, we applied the formulas used by (Ilumbe, Jaris, Lohanjola, & Habari, 2019):
Where ICs is the confirmation index (or informant consensus), Na = number of people who cited this species, and Nt = total number of people interviewed.
The local cultural importance of the plants was assessed by calculating the plant use agreement value (VAUS) used by (Ilumbe, 2010):
Ecological characterization of plants
The inventoried plant species are classified into different morphological types based on the Raunkiaer system (Raunkiaer, 1934), as adopted by (Lejoly, Lisowskis, & Ndjele, 1988) and (Menga, 2012). These types include trees (over 15 m in height), shrubs (under 7 m), small shrubs (under 2 m), lianas (climbing plants), and annual herbs (plants whose aerial and underground parts die each year). Biological types are also classified using the Raunkiaer system, extended to tropical areas. These types are geophytes (plants with buds and young leaves in the soil), therophytes (plants that persist as seeds during unfavorable seasons), caephytes (plants with small vegetative devices and persistent leaves), and phanerophytes (plants with aerial sleeping buds over 50 cm above the soil). Phanerophytes include mesophanerophytes (10-30 m tall), microphanerophytes (2-10 m tall), nanophanerophytes (0.4-2 m tall), and lynephanerophytes (woody, climbing plants). The biotope types are determined from existing floras and include primary forests, secondary forests, savannahs, ruderal areas, and cultivated species. The phytogeographical distribution is determined using the classification of Menga (Menga, 2012), based on criteria such as widely distributed species (e.g., cosmopolitan, pantropical, afro-neotropical, palaeotropical), widely distributed African species (e.g., Afro-tropical continental, Afro-Malagasy), and regional species (e.g., Sudanese, Guinean-Congolese, Zambezi).
Data processing and analysis
The data processing and analysis uses Microsoft Excel 2010 and IBM SPSS Statistics 20 as previously described (Masengo et al., 2021). Briefly, the data collected was first input into Microsoft Excel and analysed using IBM SPSS statistics. A single-variable analysis of categorical variables (social-demographic and ethnobotanical variables) was conducted to obtain descriptive statistics including relative frequencies. The Kolmogorov-Smirnov test is used to verify the normal distribution of quantitative variables. Multivariate analysis, in particular multi-correspondence analysis, is carried out to investigate the relationship between dependent variables (ethnological parameters) and independent variables (social and socio-demographic parameters).
To better compare the floras, we constructed a hierarchical ascending classification tree from a binary table of the species at the two sites, using the hclust function, according to Ward's method, based on a Euclidean distance using R software (version 4.3.2). Similarity was estimated using Jaccard's coefficient.
In silico study
Molecular docking study
Molecular docking was carried out using the seamDock server, following established protocols from previous studies. The server employs the docking_py library to prepare ligands and receptors, utilizing software like AutoDock 4, AutoDock Vina, Qvina, and Smina for docking calculations. Visualizations of the ligand-receptor interactions are facilitated by JavaScript and the NGL Viewer. A seamless framework manages the connection between the website and server. This framework, utilized in seamDock Web servers, allows for the creation of interconnected cells containing data, code, results, or web content. Cells can be synchronized and shared, with transformations defined between them. The seamless workflow operates continuously, enabling dynamic modifications, additions, and deletions of cells (Chih-Hao et al., 2002; Murail, Vries, Rey, Moroy, & Tufféry, 2021; Yu-Feng et al., 2016).
Input formatting
The preparation of the input of the ligand takes place in two steps. The first step is to convert the ligand to pdb format using the free RDKit pathformatics library. The ligand converter accepts Mol2, sdf and SMILES formats. To calculate and optimize the 3D structure of the bindings from 1D SMILES or 2D sdf/mol2 representations, the default RDKit MMFFOptimizeMolecule function parameters, the Merck MMFF94 molecular force field and up to 200 iterations are used. The pdb file of the ligand is then processed with Prepare_ligand4.py from AutoDock Tools, which assigns atomic types, calculates the atomic load, and repairs missing hydrogen atoms. All ligand torsion remains active, resulting in a pdbqt file. In order to prepare the structure of the receptor, the mass center of the receptor is first placed at a (0, 0, 0) position, and the residue of amino acids is cleaned with a filter to remove the water or ligands from the receptor and retain only protein atoms. The coordinate extension of the receptor is extracted and used to define the maximum docking box size. The protonation of protein residues is calculated using the pdb2pqr Python library, based on the Propka method at pH 7.0. Then, the autodocktools prepare_receptor4.py script is executed to calculate the types and charges of the receptor atoms and to add hydrogen atoms if there is no atom.
Docking procedure
Once ligand structure, receptor structure, docking box and docking parameters are defined, a docking can be initiated by clicking on a special button in the web form. The docking process varies depending on the selected docking software. In AutoDock, the docking grid is calculated in two consecutive steps (mgltools' prepare_gpf4.py and autogrid4 respectively). Then start MGLTools prepare_dpf4.py to prepare the Docking parameter file and use it as the input of autodock4. Vina, Smina and Qvina are simpler to perform, as grids and input parameters files do not need to be pre-calculated. All three docking software are launched directly with the receptor and ligand pdbqt files defined as inputs.
Input/Output visualization
The 3D NGL viewer is integrated into the Web page to enable interactive and comprehensive 3D visualization of different molecular structures. A 3D visualization step allows checking the calculated 3D structure of ligands and receptor inputs. All interactions between ligands and receptors are calculated by the NGL Viewer. JavaScript functions can be used to display an interactive display in the NGL scene of a docking position selected from the result table or highlight the molecular contact from the interaction table.
In this study, the major compounds of the essential oils from the seven most cited plants were selected and subjected to molecular docking analysis using enzyme RNA polymerase PB1-PB2 subunits from Influenza A Virus (PDB ID : 3A1G) as a molecular target (Sugiyama et al., 2009). These compounds are: Neral (C. citratus); Eugenol (O. gratissimum); Fenchone (T. riparia); Eucalyptol (E. globulus); (+)-Limonene (C. limon); 1, 8-Cineole (L. multiflora); and Zingiberene (Z. officinale) (Almas, Innocent, Machumi, & Kisinza, 2021; Brügger et al., 2019; Joshi, 2013; Petretto et al., 2023; Zhang et al., 2022).
Molecular dynamics simulation
Molecular dynamics (MD) was performed for 100 nanoseconds with Schrödinger LLC's Desmond software (version 2019-4) with the OPLSA force field. A 10 Å orthorhombic box was solvated using the TIP3P water model, and the system was neutralized by adding chloride ions (Ferreira, Santos, Oliva, & Andricopulo, 2021). Prior to the MD simulation, the binding of proteins and ligands was performed as the first crucial step to predict the static view of the binding position of molecules in the active site of proteins (Hildebrand, Rose, & Tiemann, 2019). MD simulations, which incorporate Newton's classical motion equations, generally simulate atomic movements over time and predict the binding state of ligands in physiological environments (Rasheed et al., 2021). The ligand-receptor complex was preprocessed by the Maestro Protein Preparation Wizard, which included optimization, minimization and, if needed, filling missing residues, as well as a system created via the system builder tool. The TIP3P (Three-Point Transferable Intermolecular Interaction Potential) solvent model is based on an orthorhombic box with a temperature of 300 K, 1 atm pressure and a force field of OPLS_2005 (Shivakumar et al., 2010). The model is neutralized and simulates physiological conditions using a counter ion and a 0.15 M sodium chloride. The models are loosened before simulation, and the paths are stored for inspection after each 100 ps. The equilibration of the protein (PDB ID: 3A1G) and bound ligand (eugenol) was monitored by tracking fluctuations in their respective RMSDs throughout the simulation. In addition, the ‘extendedness’ of the ligand was assessed by computing its radius of gyration (rGyr) at each simulation step, where rGyr is equivalent to its principal moment of inertia (Matondo et al., 2022).
Ethical considerations
The study protocol was approved by the Ethics Committee of the Life Sciences Department at the University of Kinshasa (ethical clearance certificate number EPHYMED/015/CDB/2023). The study followed the principles laid down in the Helsinki Declaration to ensure that respondents provided their free and informed consent. Furthermore, the study fully adheres to the confidentiality and ethical rules of the Democratic Republic of the Congo, as well as to the regulations relating to access and benefit sharing (ABS) in relation to the use of plant genetic resources. The respondents were explicitly informed that their participation in the survey was entirely voluntary and free of any form of coercion. They also confirmed that the results of the study would be shared with them through an open-access article that local leaders would be able to distribute (Masengo et al., 2021; Mongeke et al., 2018).
RESULTS AND DISCUSSION
Sociodemographic profile of respondents
Table 1
Socio-demographic characteristics of the respondents
A total of 104 people participated in the survey, 54 (52%) and 50 (48%) were men and female respectively (Table 1). This result differs from the results of Sassa (2013) and Menga et al. (2022), in which women dominate, but which contradicts Bokulu (2019) and emphasizes men's predominance. Among the respondents, young people aged 18 to 35 were the majority (52.9%), followed by adults (36 to 50 years: 31.7%) and elderly people (15.4%) (Table 1). This age distribution follows the normal distribution of Kolmogorov-Smirnov (p = 0.9983>0.05). The dominance of young people in knowledge transmission is proof that they are now responsible for the problem and will be able to take over the transmission of naturalistic knowledge. Half (50%) of the respondents had secondary education. Illiterate people make up 21.2% of the sample, and primary school-aged people make up 20.2%. University graduates are the least represented (Table 1). The results show that 104 participants were 18 years old at the minimum, 60 years old at the maximum, 38.22 years on average, and 14.15 years on average. The Kolmogorov–Smirnov test (dof 10.4 = 0.2319, p=0.000) and the Shapiro–Wilk test (dof 10.4 = 0.896, p=0.000) show that the age distribution of the respondents does not match the normal distribution of the population in the area of the survey (p<0.05).
Regarding marital status, Table 1 also indicates that married individuals were the most numerous, followed respectively by single and divorced individuals.
Floristic and ecological characteristics
Distribution of taxa
The results of ethnobotany survey on anti-influenza medicinal plants traditionnaly used in Ibi villages revealed 40 plant species divided into 36 species, 28 families, 17 species and 6 classifications. The Malvidae clan has four orders, 10 families and 13 species. The Zingiberaceae family is best described in three species (Aframomum alboviolaceum, A. melegueta, Zingiber officinale). Details are provided in Table 2.
Table 2
Floristic list and ecological characteristics of species
Legend: TM: Morphological types; TB: Biological types; DP: Phytogeographical distribution; MsPh: Mesophanerophytes; McPh: Microphanerophytes; NPh: Nanophanerophytes; Phgr: Climbing phanerophytes; Chd : Chamaephytes; Gb: Bulbous geophytes; Grh: Rhizomantose geophytes; Thd: Erect therophytes; F: Forest; Sav: Savanna; Rud: Ruderal; Cult: Cultural; PR: Multiregional; Pan: Pantropical; At : Afro-tropical; Pal: Palaeo-tropical; CGC: Centroguinean; Guin: Guinean T: Trees; Shr: Shrubs; Subs: Subshrubs; Lia: Lianes; Ha: Annual herbs; PH: Perennial herbs.
Ecological characteristics of plants
Biological types
Figure 2A illustrates the spectrum of biological types. Phanerophytes predominate (77%), followed by geophytes (10%) and chamaephytes (8%). These results corroborate those of (Menga, Amogu, Makwela, Bikandu, & Lukoki, 2022; Ngbolua et al., 2019; Sassa, 2013). Phanerophytes are woody perennial plants that are rich in secondary metabolites due to their efficient anatomical structures (Bokulu, 2019).
Morphological types
Analysis of the morphological types of the species recorded shows the preponderance of shrubs (33%) and trees (28%), with the other categories less well represented (Figure 2B). These results corroborate those of (Menga et al., 2022; Ngbolua et al., 2019; Sassa, 2013), but with a ratio of 30% trees and 21% shrubs.
These results demonstrate the impact of woody plants in the treatment of traditional influenza-related pathologies, according to (Ngbolua et al., 2016), woody species have a high alkaloid content compared with herbaceous plants. In addition to the fact that the perennial nature of these plant species guarantees the availability and sustainability of the raw material, these woody plants contain anatomical production and reserve structures such as secretory canals, secretory pockets and more efficient latex cells that enable them to produce and accumulate secondary metabolites responsible for curative power.
Types of leaf size
Analysis of leaf types shows a clear preponderance of mesophyllous species (67%), with the other categories less well represented (Figure 2C). This high level of representation is considered linked to the nature of the environment in which a large proportion of the species inventoried are found, since they come from a forest environment and therefore benefit from very low light levels, which are favourable to the development of mesophyll species (Tshituka, 2015).
Types of diaspora
Analysis of diaspore types (Figure 2D) highlights the prevalence of sarcochorous species (67%) and, to a lesser extent, sclerochorous species (15%) and ballochorous species (10%). Sarcochorous species have fleshy, edible fruits whose juicy pulp is highly prized by rodents, birds, and humans, ensuring widespread dissemination of diaspores. However, this dissemination occurs over short distances because the diaspore is relatively heavy and most of the animal consumers involved (except for humans) prefer to eat it on the spot rather than transport it over long distances. As a result, dispersal occurs from near to near, over short distances (Menga et al., 2022).
Habitat types
Habitat types are shown in Figure 2E. This figure shows that 46% of the species are cultivated and 26% come from forest and savannah, respectively. These results are similar to those of (Sassa, 2013) and (Menga et al., 2022). The predominance of cultivated species in the medicinal flora of the IBI village and surrounding area is evidence of the integration of medicinal plants into the agricultural sector and constitutes a participatory and sustainable management strategy that will make it possible to perpetuate phytomedicinal resources. This is an encouraging solution for the long-term survival of the species. However, it would be better to put these observations into perspective, given that most cultivated species are exotic (Menga et al., 2022).
Chorological types
Analysis of chorological types shows the prevalence of Pantropical species (43%), followed by Guineo-Congolese species (33%); the other categories are less well represented (Figure 3). The predominance of pantropical species in this study show that the local flora has undergone some contact with flora with eastern and southern affinities, through the cultivation of exotic plants (Mawunu et al., 2023; Menga et al., 2022).
Figure 2
Ecological characteristics of inventoried plants (A. Biological types ; B. Morphological types ; D. Leaf types; D. Types of diaspora; E. Habitat types).
GC = Guineo-Congolese, Pal = Palaeotropical, AA = Afro-American, AM = Afro-Malagasy, GC-SZ= Guineo-Congolese Sudano-Zambezian, Pan= Pantropical, PR = Multi-regional.
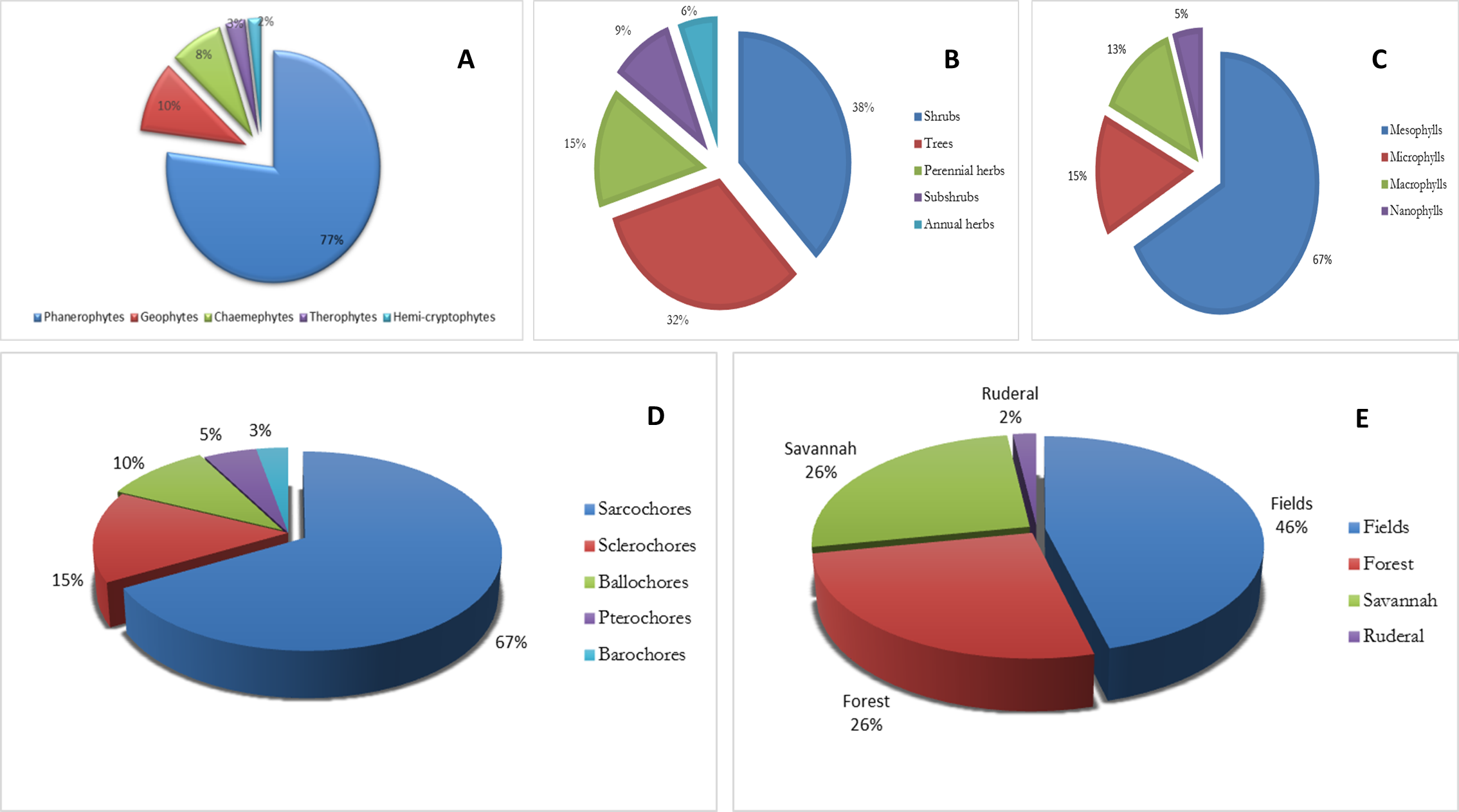
Ethnobotanical characteristics of plants
Informant consensus and cultural importance
This ethnobotanical survey of 104 people identified 40 medicinal plants used to treat influenza, which was cited 281 times and were involved in 64 medicinal recipes. Table 4 shows the cultural importance of each plant inventoried. The table shows that the most frequently cited species with the highest cultural importance are: C. citratus (16.7% cited; VAUs = 0.30), O. gratissimum (12.8% cited; VAUs = 0.23), T. riparia (11.7% cited; VAUs = 0.21), E. globulus and C. limon (respectively 7.1% cited; VAUs = 0.13), Z. Officinale and L. multiflora (respectively 6.4% cited; VAUs = 0.12).
These results differ slightly from those of (Menga et al., 2022) who inventoried 42 medicinal plants used to treat influenza in the urban neighbourhoods of Mont-Ngafula commune in Kinshasa. The most frequently cited plants were O. basilicum (18.9%), C. ambrosioides (11.5%), C. citratus (7.9%), T. riparia (6.8%), C. limon (6.4%), M. indica (5.6%), P. americana (4.8%), D. edulis (4.1%), and B. patula (3.7%). However, there is no great similarity between the two floras (Jaccard's coefficient = 0.464), although 26 species are common to both, including A. melegueta, A. cordifolia, C. limon, C. sinensis, C. citratus and D. edulis, E. globulus, H. acida, L. multiflora, M. indica, M. whitei, M. morindoides, M. oleifera, N. tabacum, O. basilicum, O. gratissimum, P. americana, P. guayava, S. longipenduculata, T. riparia, Z. officinale. The flora of anti-influenza medicinal plants at Mont-Ngafula contains a higher proportion of exotic and cultivated species than at Ibi-village on the Batékés plateau, as shown in the Figure 4.
Figure 4
Dendrogram of Euclidean distance between the different surveys (Ward's method), (Legend: in green: species common to both floras, in red: species exclusive to Ibi, in blue: species exclusive to Mont-Ngafula).
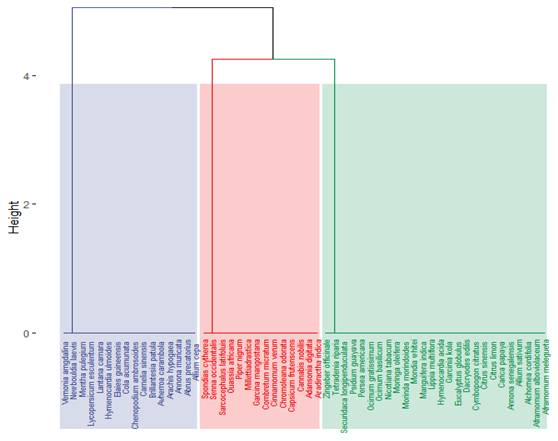
Table 4
Use agreement value of plants inventoried
Parts of plants used in medicinal recipes
The parts of the species used in the recipes to treat influenza are mainly the leaves (80% cited), followed to a lesser extent by the fruit (11% cited), and with the other organs cited least often (Figure 5). These results are similar to those of (Menga et al., 2022), who obtained almost similar proportions, i.e. 75% of citations for leaves and 13% for fruits. Similar observations were also made by (Lautenschläger et al., 2018; Mawunu et al., 2022; Sassa, 2013) in Angola; (Bikandu, 2012; Djoza et al., 2023; Liyongo et al., 2023) in the DRC. The high frequency of use of leaves is partly explained by the ease and speed of harvesting (Bitsindou, 1996; Ngbolua et al., 2019), but also by the fact that most secondary metabolites are accumulated in them. In addition to this, the use of leaves and fruits in phytotherapy is a non-destructive practice, allowing sustainable use of the plant, if harvesting is carried out non-invasively.
Preparation of medicinal recipes
The proportions of the different methods of preparation of anti-influenza medicinal recipes are shown in Figure 6. Figure 6 shows a high use of decoction (82%) and, to a lesser extent, infusion (7%); the other preparation methods are less well represented. These results corroborate those of (Bikandu, 2012; Menga et al., 2022; Sassa, 2013). The prevalence of decoction can be explained by the fact that this method of preparing recipes makes it possible to disinfect the raw plant material (Mawunu et al., 2022; Tahiri, Benaabidate, Nejjari, & Benbrahim, 2012), and to collect the most active ingredients and attenuate or cancel out the toxic effects of recipes (Ngbolua et al., 2019). Infusion, otherwise, prevents thermal denaturation of the active ingredients (Ngbolua et al., 2019).
Composition of medicated recipes
In most of the influenza recipes in the region studied, plants were used in combination with other plants (multispecific recipes, 73%) and to a lesser extent alone (monospecific recipes, 23%). These results differ from those of (Menga et al., 2022), who found that monospecific recipes predominated. According to (N’guessan, Bi, & Koné, 2009), the use of multispecific recipes may entail health risks for patients. In fact, plant-based mixtures, when mismatched, are dangerous and toxic. In Africa, where the majority of the population depends on herbal medicine, it is reported that around 30% of fatal accidents are generally linked to combinations of medicinal plants. These results differ from those of (Menga et al., 2022) where monospecific recipes predominate.
Use of medicinal recipes
The proportions of the different forms of use of anti-influenza medicinal recipes are shown in Figure 7. Most recipes are used in the form of syrups (57%), and sometimes as inhalations (10%).
Methods of administering drug recipes
Recipes are administered are shown in Figure 8. It can be seen that the oral route (77%) is the most commonly used for administering flu recipes, followed by the steam bath (14%).
Physical state of consumption of recipes and opinion on treatment efficacy
Most of the plants used to combat influenza in Ibi-villages are consumed in their fresh state (93%), while the dry state accounts for barely 7%. Approximately 62% of those surveyed said that anti-influenza treatment based on plants gave a complete cure, 34% thought that the cure was partial, while only 4% thought that the use of plants had no flu at all. There is currently a great deal of experimental evidence demonstrating the anti-flu activity of medicinal plants (Wang et al., 2006; Pleschka et al., 2006; Rajasekaran et al., 2013; Mohamed et al., 2020).
From Figure 9, it can be observed that only the mode of recipe administration has been influenced by the sociodemographic parameter "marital status." However, it should be noted that the respondents can be grouped into two categories based on ethnomedical practice: the first group consists of those who prescribe, recipes based on the condition of the plant and the parts used (axis 1), and then those who prescribe recipes based on the mode of administration, preparation method, and form of use (Figure 10).
Figure 12; Figure 11, respectively, provide the energetic values of complexation (thermodynamic stability of complexes formed between the receptor and chemical compounds from various essential oils) and the hierarchical classification of these compounds based on thermodynamic stability.
Figure 11
Thermodynamic stability of the complexes formed between the receptor and the major chemical compounds of the selected plant essential oils.
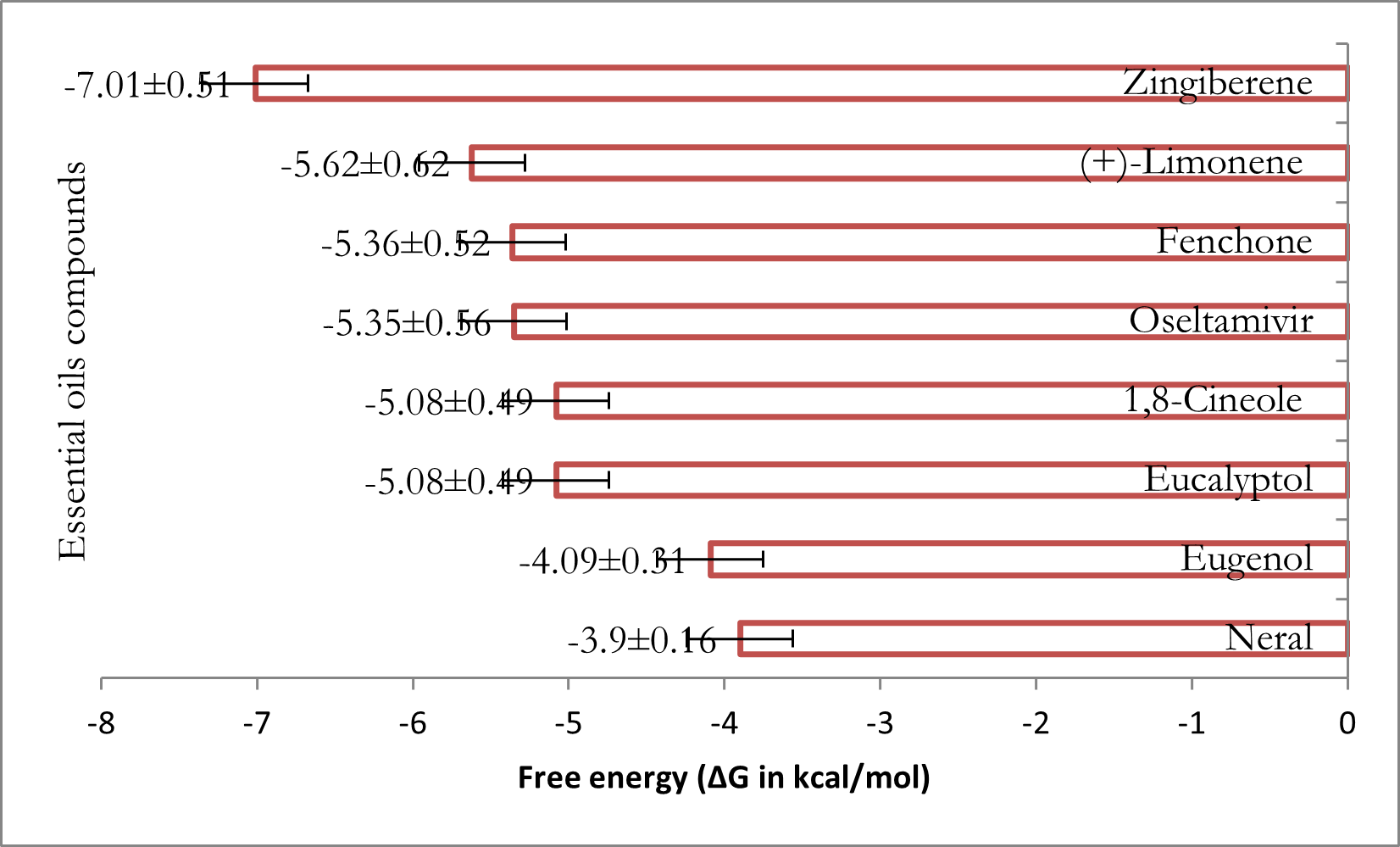
It can be deduced from this figure that the selected compounds form a thermodynamically stable complex and are divided into five classes, namely: Class 1: Neral (-3.9±0.16 kcal/mol) and Eugenol (-4.09±0.31 kcal/mol); Class 2: Fenchone (-5.36±0.52 kcal/mol) and Oseltamivir (-5.35±0.56 kcal/mol); Class 3: Eucalyptol (-5.08±0.49 kcal/mol) and 1,8-Cineole (-5.08±0.49 kcal/mol); Class 4: (+)-Limonene (-5.62±0.62 kcal/mol); and Class 5: Zingiberene (-7.01±0.51 kcal/mol). Only two compounds, Neral [N9(D) OD1; R8(D) NH2], and Eugenol [N9(D) OD1; N9(D) ND2; R8(D) NE; R8(D) NH2], form two and four hydrogen bonds with the receptor, respectively, while the positive control forms six [C692(A) O; C693(A) SG; T24(B) O; V25(B) O; V25(B) O; C693(A) SG].
The RNA-dependent RNA polymerase of the influenza virus (Figure 14; Figure 13) is a versatile heterotrimeric complex that employs a 'cap-snatching' mechanism to generate viral mRNA. This process involves the cleavage of host cell mRNA to generate a cap-containing oligonucleotide, which can then be elongated using the viral genomic RNA as a template. The inhibition of it by both compounds (eugenol and neral) is evidence of the potential anti-influenza activity of these aromatic plants.
Figure 13
Crystal Structure of RNA polymerase PB1-PB2 subunits from Influenza A Virus (Sugiyama et al., 2009).
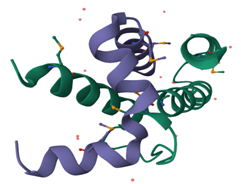
Figure 14
Structure and physicochemical properties of Neral (A) and Eugenol (B); RNA-dependent RNA polymerase complex with neral (C), eugenol (D) and oseltamivir (Positive control) (E)
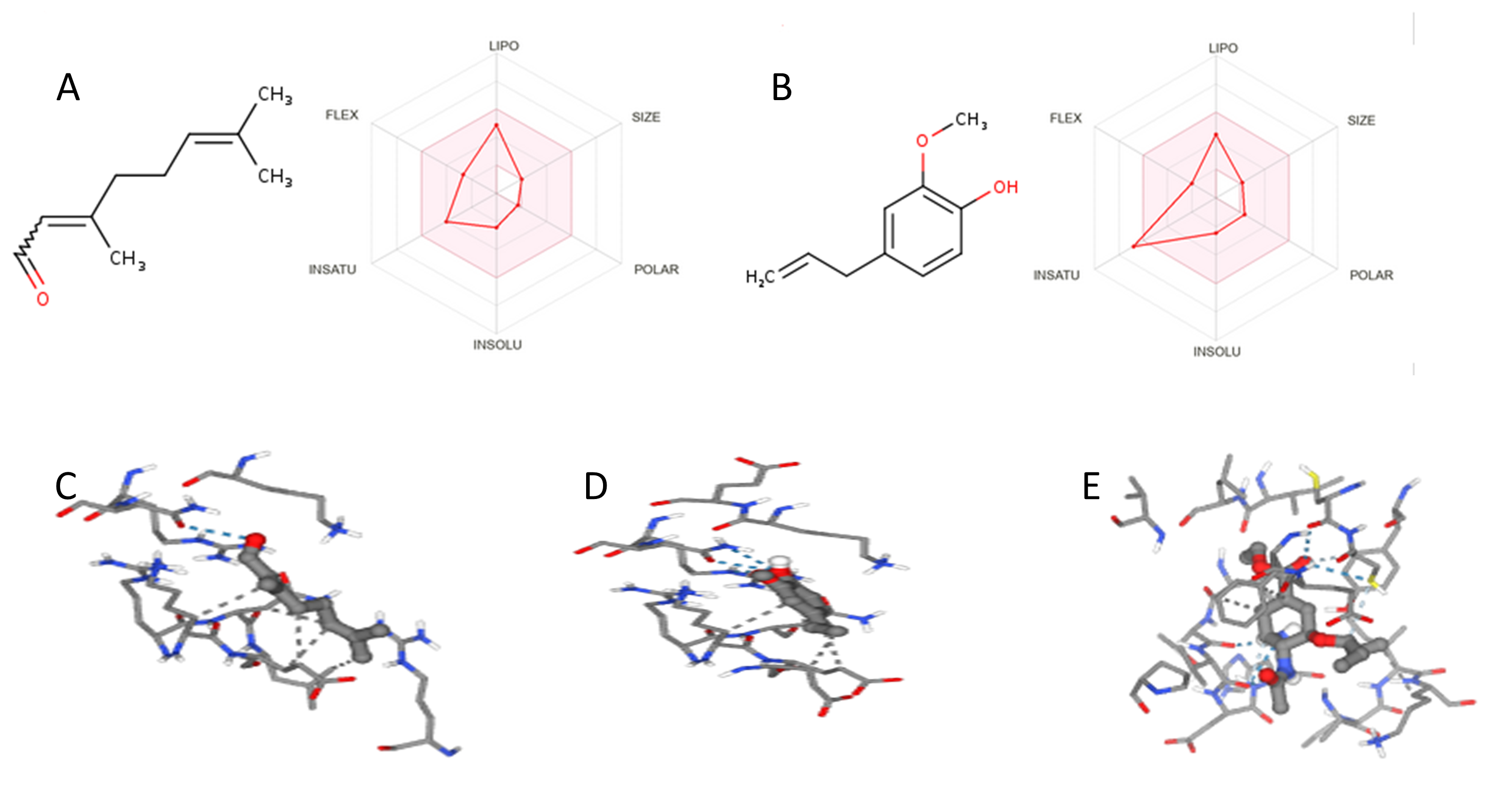
Molecular docking and molecular dynamics (MD) were employed in this study as tools to predict the antiviral properties of the selected compounds. Molecular docking predicts the interaction of an active compound with a specific biological target, providing insights into the potential mechanism of action. On the other hand, MD simulates the movement of atoms and molecules over time, offering information about the stability of molecular interactions. This approach allows for the simulation of a compound's behavior within a complex biological environment. In this study, compounds considered stable (ΔG < 0) and forming at least one hydrogen bond (eugenol and neral) underwent a thorough analysis to estimate their molecular electrostatic potential. The bioactive compound shows a high molecular electrostatic potential (MEP: eugenol) was selected for MD. A high value of MEP indicates the presence of strongly charged regions, influencing the molecule's ability to interact with its biological target. Strongly positive regions attract negatively charged regions of surrounding molecules, and vice versa, playing a crucial role in molecular interactions such as binding with biological receptors. The study results indicate that eugenol possesses a region delimited by oxygen atoms 2 and 4 with a high electrostatic potential, suggesting a highly reactive area. Meanwhile, the region between hydrogen 22 and hydrogen 17 are electronically repulsive (Figure 15a). In comparison to eugenol, neral is potentially less electrostatic (Figure 15b) but exhibits equivalent electronic repulsion on the opposite side. It is noteworthy that the area delimited by oxygen atom 4 and carbon atom 1 shows a high electrostatic potential, indicating a highly reactive region of neral. On the other hand, the region between carbon 27, hydrogen 13, and hydrogen 14 are electronically repulsive.
The RMSD (Root Mean Square Deviation: Figure 16a) and RMSF (Root Mean Square Fluctuation: Figure 16b) are two important measures used in molecular dynamics to assess the stability and flexibility of molecular structures over time. RMSD calculates the root mean square deviation between the positions of atoms in a molecular structure during simulation compared to a reference structure. It is noteworthy that the formed complex remains stable only between 90 and 100 ns, as indicated in the figure below. Indeed, during this time interval, the ligand maintains a low deviation from the receptor.
Figure 16
A) Root Mean Square Deviation plot obtained for the 3A1G-Eugenol complex; B) Root Mean Square Fluctuation plot obtained for 3A1G-Eugenol complex; C) Protein-ligand contact plots ; D) Ligand property trajectory.
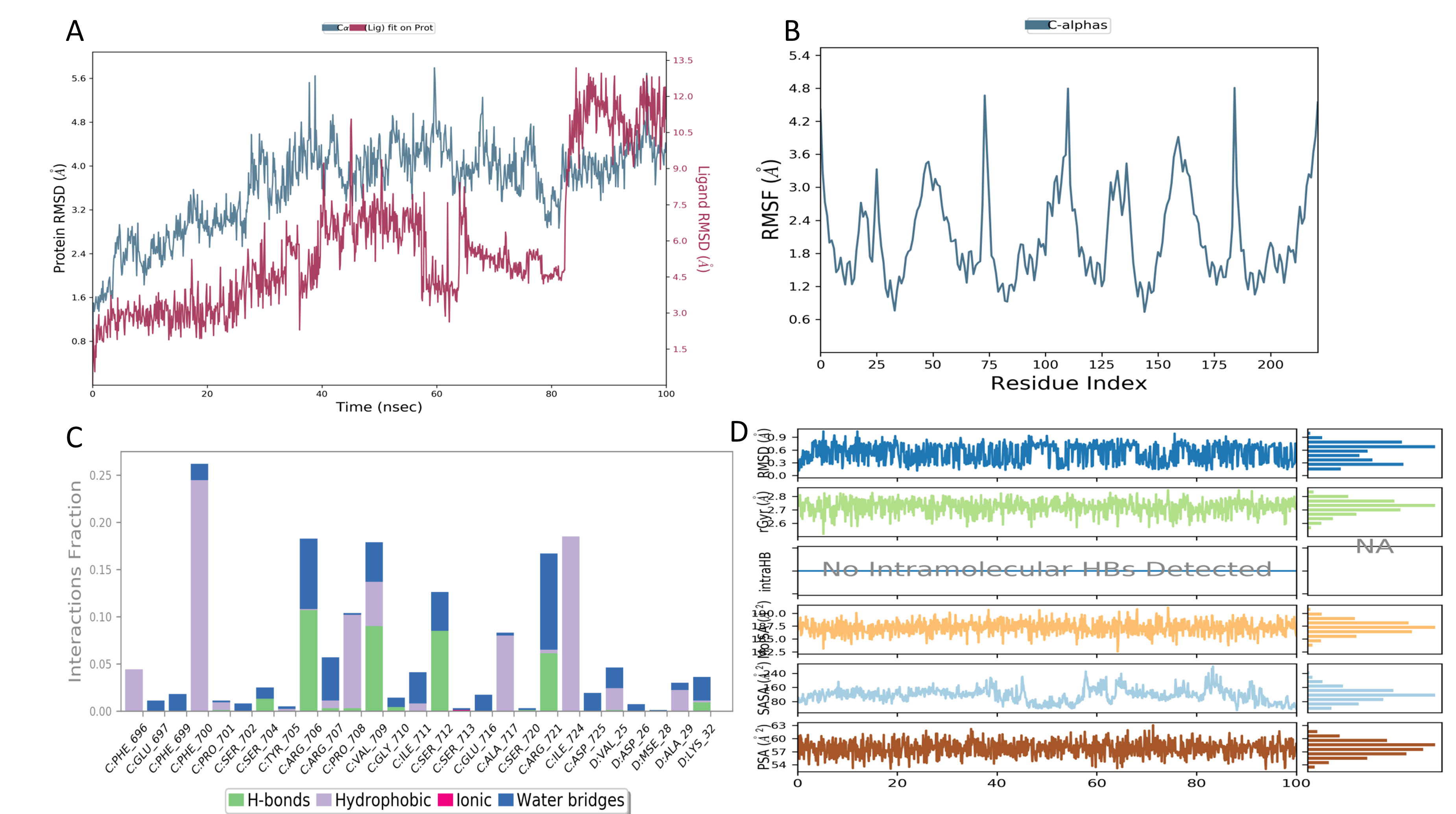
On the other hand, RMSF (Root Mean Square Fluctuation) measures the amplitude of fluctuations in atomic positions relative to their mean position during the simulation. The results of the current study indicate a high RMSF for a specific group of atoms, suggesting significant flexibility or mobility in that region (Figure 16b).
Approximately 250 residues of 3A1G were calculated using the Root Mean Square Fluctuation (RMSF) to explore the flexibility of each residue and its fluctuation during the simulation period as indicated in the Figure 16b. A consistent RMSD (between 90 and 100 ns) with a high RMSF (>4) indicates that the protein receptor maintains its overall structure, but specific parts fluctuate significantly, indicating what is referred to as local flexibility.
The Figure 16c allows for the identification of interactions that stabilize the complex. These interactions fall into three types: hydrophobic interactions (bonds), hydrogen bonds, and water bridges.
In general, nearly 300 amino acid residues are involved in the interaction with the ligand. However, specifically, the amino acids Arg_706, Val_709, Ser_712, and Arg_721 interacted with the ligand through hydrogen bonds. Hydrogen bonds play a key role in protein-ligand interactions and protein folding; hence, they are crucial to consider in drug design (Abdalla, Eltayb, El-Arabey, Singh, & Jiang, 2022; Boufissiou et al., 2022).
To assess the stability of the selected compound, its properties were analyzed by calculating RMSD, molecular surface area (MolSA), solvent-accessible surface area (SASA), gyration radius (rGyr), and polar surface area (PSA) (Figure 16d). The RMSD is a measure of the deviation of atomic positions from a reference structure over time. Molecular surface area (MolSA) represents the total surface area of a molecule, providing information about the overall size of a molecule and its interactions with other molecules or surfaces. Solvent-accessible surface area (SASA) measures the surface of a molecule that is accessible to solvent molecules, offering insights into interactions between a molecule and its environment. Changes in SASA can indicate structural modifications during processes such as binding or ligand release. Gyration radius (rGyr) is a measure of the average size of a molecule, calculated by considering the positions of atoms relative to the center of mass. It provides information about the compactness of a molecule and how it folds or unfolds. Polar surface area (PSA) measures the surface of a molecule occupied by polar atoms, helping predict the molecule's solubility as polar molecules tend to interact more easily with water molecules (Abdalla et al., 2022; Boufissiou et al., 2022).
The various properties of the ligand analyzed, except for SASA, exhibited low fluctuations at the beginning of the simulation but gradually reached equilibrium and remained constant throughout the simulation. This confirmed the stability of the ligands in the protein's active site. However, it is important to note that no intramolecular hydrogen bonds were detected.
Table 5
Off-target activity predictionand the ADMET profile of Eugenol
Eugenol, the biologically active principle of selected plants, is a natural compound with several remarkable pharmacobiological properties. It is recognized for its analgesic, anti-inflammatory, antimicrobial, and antioxidant effects. These properties make it a promising candidate for the treatment of pain, inflammation, infections, and as an antioxidant agent. It is also well known for its beneficial effects on digestion. However, it may present toxicological risks, including acute toxicity at high doses, skin allergic reactions, and adverse effects on reproduction (Juergens, 2014; Prashar, Locke, & Evans, 2006; Viuda-Martos, Ruiz-Navajas, Fernández-López, & Pérez-Alvarez, 2008). The prediction of probable off-target activity and the pharmacokinetics of eugenol using the ProTox-II web tool (Umar et al., 2022) shows that the predicted LD50 is approximately 1930 mg/kg (predicted toxicity Class: 4). This compound is likely to interact with the Androgen receptor, Amine oxidase A, and Prostaglandin G/H synthase 1. From a pharmacokinetic standpoint, this compound is predicted to be potentially neurotoxic and can cross the blood-brain barrier while inhibiting the cytochromes CYP2C19 and CYP2C9 (Table 5 ). Bioinformatic prediction also shows that eugenol is biologically active against five cancer cell lines: PC-6 (small cell lung carcinoma), MDA-MB-453 (breast adenocarcinoma), NALM-6 (adult B acute lymphoblastic leukemia), MeWo (melanoma), and RPMI-8226 (multiple myeloma), and against three non-tumor cell lines: WI-38-VA13 (embryonic lung fibroblast), HaCat (keratinocyte), and IMR-90 (embryonic lung fibroblast). Furthermore, it has also been demonstrated that eugenol is a promising candidate for combating various viruses. Laboratory studies have shown that eugenol has the ability to inhibit the replication of several viruses, including herpes simplex virus, influenza virus, and hepatitis C virus and SARS-CoV-2 (Manivannan et al., 2022).
The results of molecular dynamics validate those of molecular docking. Thus, by combining the ethnobotanical approach with molecular docking and molecular dynamics, this study has demonstrated the potential efficacy of C. citratus and O. gratissimum against influenza at the molecular level. These two plants could be used therapeutically to combat influenza in the DRC.
This study thus strongly emphasizes the need to protect traditional knowledge because it is obvious that some of the owners of such knowledge are older (47.1%), and the risk of losing valuable information is high. In order to ensure the fair and ethical distribution of this knowledge and in accordance with the principles of access and beneficial distribution, the findings of the study must be returned to traditional knowledge owners through a large-scale awareness campaign. In addition, in order to expand the scope of research to other regions, close collaboration with local organizations, especially in the field of the efficacy and safety of plant products, is important. The establishment of a comprehensive and harmonised plan for ethnobotany research in the DRC will facilitate the documentation of traditional knowledge for knowledge owners and scientific communities. This is the first study in this region on respiratory diseases such as influenza to date.
Medical facilities, especially in the DRC, play an important role in the management of diseases in poor areas within the framework of universal health care. Medical facilities are often affordable and free, so people with low incomes can afford expensive medical services (economic access). In many parts of Africa, knowledge of plant medicinal properties is passed down from generation to generation. Traditional healers and local herbalists have extensive experience in treating many diseases using these plants.
These plants tend to grow naturally and are easily available to local communities, reducing the need to spend money on imported drugs. The use of medicinal plants is deeply rooted in the culture and identity of many communities, contributing beyond physical health to the spiritual and mental well-being of individuals. It should be noted that rural medical infrastructure is limited. In this respect, medical plants can complement modern medicine by providing treatments when other options are not available (complementing modern medicine). The risk of serious side effects is also lower than some synthetic drugs. This reduces the risk to patients, especially in areas where emergency medical services are limited. However, medical use requires detailed knowledge to avoid unwanted side effects and potential drug interactions. Furthermore, scientific research is needed to verify the effectiveness and safety of these treatments. In Africa, in the context of universal health coverage, traditional knowledge of plants must be combined with modern medical practices to ensure that everyone receives comprehensive and high-quality health care.
CONCLUSIONS AND SUGGESTIONS
The ethnobotanical study of plants used to treat influenza carried out in Ibi-village on the Batékés plateau, has achieved all its objectives. A total of 40 plants used in the traditional care and treatment of influenza were identified, including C. citratus, O. gratissimum, T. riparia, E. globulus, C. limon, Z. officinalis and L. multiflora, all of which are particularly well known to the local population and widely used to treat flu-like illnesses, either alone or in association with other species. Often, it is the leaves that are used to prepare the drugs, which are often made by decoction. The majority of these plants are shrubs and trees, most of which are cultivated, making them inexpensive, accessible, and effective. Influenza and cough are the illnesses for which traditional recipes are best suited. The results of molecular dynamics and molecular docking combined with the ethnobotanical approach have demonstrated the potential efficacy of C. citratus and O. gratissimum against influenza at the molecular level. These two plants could be used therapeutically to combat influenza. It is therefore desirable to formulate a phytomedicine based on these two plants in order to verify or test its efficacy and its toxicity in vitro and in vivo; to carry out phytochemical studies in order to identify the chemical compounds and isolate the molecules responsible for treating influenza.
LIMITATION OF THE FINDINGS
This study has limitations, including ethnobotanical biases due to the variability of local knowledge, and the reliability of the collected data depending on the accuracy of the informants. These limitations underscore the need for further studies (in vitro and in vivo) to confirm the efficacy and safety of eugenol and eugenol-rich extracts.
ETHICS APPROVAL AND CONSENT TO PARTICIPATE
This study has been conducted under the provisions of the Nagoya Protocol on Access to Genetic Resources and the Fair and Equitable Sharing of Benefits Arising from their Utilization of the Convention on Biological Diversity. Oral Prior consent was obtained from each participant. This study does not contain any experimente (s) on humans and animals. This study has been conducted under the provisions of the Nagoya Protocol on Access to Genetic Resources and the Fair and Equitable Sharing of Benefits Arising from their Utilization to the Convention on Biological Diversity. During the ethnobotanical data collection from informants a prior oral consent was taken. Consent for publication: Not applicable-this manuscript has no personal data from the authors. Availability of data and materials: The original data is presented in the article. There is no supplementary data. The raw data without the names of informants can be provided by authors.
Author contributions
PMM conceived and designed the study. GKG and CMA carried out data collection, integrated the inventory, and performed the analysis, as well as contributed to manuscript writing. PMM, MM, PTM, VM and NKN identified the plants, reviewed, and edited the manuscript. All authors have read and approved the final published version.