INTRODUCTION
There has been a growing interest in natural products due to their phytochemical diversity, low toxicity, and diverse pharmacological properties (Shahrivari, Alizadeh, Ghassemi-Golezani, & Aryakia, 2022). Plants have historically been used as a valuable reservoir of bioactive molecules, intensively explored for pharmaceuticals and functional ingredients for various health conditions and food preservation (Pandey, Pradhan, Joshi, & Adhikari, 2022). Among these, the Melaleuca cajuputi (commonly known as Gelam or Cajuput tree) stands out as a plant species with attractive pharmacological properties (Bua et al., 2020; My, 2020; Xu et al., 2020). M. cajuputi is a plant species that belongs to the Myrtaceae family. It is indigenous to Southeast Asia, specifically Malaysia, Thailand, Indonesia, and the Philippines. Different M. cajuputi parts have been used in folk medicine to manage digestive ailments, cough, anxiety, stress, and skin infections (Isah et al., 2022). Previous studies have documented diverse bioactive components from medicinal plants with promising therapeutic potential (Alfred et al., 2022). These compounds include polyphenols, terpenes, glycosides, alkaloids, and phenolics (Anza et al., 2021; Mera, Falconí, & Córdova, 2019). Antioxidants are essential in reducing oxidative stress, a factor associated with numerous chronic conditions, such as cardiovascular diseases, cancer, and neurodegenerative disorders, thereby neutralizing harmful free radicals and protecting against cellular damage (Yang et al., 2021). Interestingly, plant-based compounds have been shown to exhibit promising antioxidant activities (Isah et al., 2022). Tyrosinase is an enzyme responsible for melanin production, and its overactivity can lead to hyperpigmentation disorders, such as melasma and age spots. The primary therapeutic approach for these disorders involves inhibiting tyrosinase activity (Liu et al., 2021). Hydroquinone and kojic acid, for example, are well-established and effective anti-tyrosinase agents. However, these compounds have been associated with many adverse effects, including skin cancer, inflammation, dermatitis, and melanocyte destruction. Thus, exploring alternative agents from natural products is imperative (Jia et al., 2023). On the other hand, the enzyme acetylcholinesterase is essential in the pathogenesis of neurodegenerative disorders. Hence, suppressing the activity of these enzymes has been linked to enhanced cognitive performance and holds significance in managing Alzheimer’s disease (Jia et al., 2023). Identifying alternative anti-cholinesterase agents with improved pharmacological profiles from natural sources is necessary to supplant synthetic compounds associated with numerous adverse effects (Saber et al., 2021). Nevertheless, in addition to investigating the biological activities of plant extracts and compounds, safety evaluation is critical to verify that these plant extracts do not cause detrimental effects when applied topically or administered systematically (Sintayehu et al., 2022). Furthermore, certain plant-based compounds are being recognized for their antioxidant, antimicrobial, and enzyme inhibition properties. This phenomenon has increased researchers' focus on these compounds in drug discovery and development (Eyupoglu et al., 2022). Hence, this research examines the antioxidant, anti-tyrosinase, anti-cholinesterase, and toxicological properties of the methanolic leaf extract and its fractions from M. cajuputi leaf. Furthermore, the binding affinities of the active compounds towards the target enzymes were investigated using molecular docking studies.
MATERIALS AND METHODS
Sample collection and identification of plant materials
Melaleuca cajuputi leaf samples were collected from Bachok District (6°0’N 102°22’E) Kota Bharu, Kelantan, Malaysia. Plant materials were identified at the International Islamic University of Malaysia (IIUM), and the specimen number (PIIUM 0304) was deposited at the IIUM herbarium unit. The plant samples were dried in the oven at 45℃ and then ground into a fine powder using an electric grinder. The samples were stored in a freezer at 4°C until needed for the experiments.
Preparation of crude methanolic extract
Fifty grams of powdered leaves from the M. cajuputi plant were immersed in 500 mL of methanol at room temperature for 72 hours. The mixture was stirred using a magnetic stirrer to ensure homogeneity. The sample was filtered using Whatman No. 1 filter paper, followed by solvent evaporation using a vacuum evaporator at 50°C under reduced pressure. This process yielded the crude methanolic extract of M. cajuputi leaf (Uba et al., 2022).
Fractionation of the crude methanolic extract
The methanolic extract (10 g) was subjected to fractionation using column chromatography on silica gel 60 (0.040-0.063 mm). The column was sequentially eluted using n-hexane, ethyl acetate (EtOAc), and methanol (MeOH). The polarity of the eluting solvent mixture was increased from 100% hexane by gradually increasing the volume of ethyl acetate (EtOAc) by 10% increments up to 100%. The solvent mixture was subsequently changed to a combination of EtOAc and MeOH, with an initial ratio of 10% MeOH and 90% EtOAc. This ratio gradually increased until reaching 100% MeOH, resulting in twenty-two distinct fractions. The fractions with similar TLC profiles were pooled into four Melaleuca fractions (MF1, MF2, MF3, and MF4). These fractions were screened for bioassays (Erhabor, Aderogba, Erhabor, Nkadimeng, & Mcgaw, 2021).
DPPH assay
The antioxidant activities of MCME and fractions were measured using the DPPH assay, as described by Pandey et al. (2022). Methanol (100 mL) was used to dissolve DPPH (3.9432 mg) to prepare a 100 mM DPPH solution in methanol. Various concentrations of the extract and fractions were prepared, spanning from 15.625 µg/mL to 1 mg/mL. Methanol and ascorbic acid were used as the blank and positive controls, respectively. The mixture was kept in the absence of light at room temperature for 30 min, absorbance was measured at a wavelength of 517 nm. The data was presented as IC50 values and expressed as milligrams of ascorbic acid equivalent per gram of extract (mg AE/g extract). The estimation of DPPH scavenging percentage was conducted using the following equation.
DPPH Radical Scavenging
The variable A represents the absorbance value of the control, while the variable B represents the absorbance value of the test. The IC50, which represents the inhibitory concentration at which absorbance reaches 50%, was determined through a regression analysis of the average percentage of DPPH scavenging.
Tyrosinase inhibition assay
All the plant samples were evaluated for tyrosinase inhibition, as described by Alfred et al. (2022). Each plant extract and fractions (40 µL) of different concentrations ranging from 1000 µg/mL– 15.625 µg/mL, phosphate buffer (80 µL at pH 6.8, 100 mM), and tyrosinase enzyme (40 µL) from mushroom (25 KU; 1mg= 1881 units) were added in 96-well microplate flat bottom and kept for 10 min at 25℃. A L-Dopa (l-3,4-dihydroxyphenylalanine) (40 µL) was added, and the mixture was kept in the dark for 30 min. Subsequently, an ELISA plate reader (BIO-RAD) was used to take the absorbance at 515 nm. The reference compound was kojic acid, while dimethyl sulfoxide (DMSO) was the blank control. The formula for calculating tyrosinase inhibition is as follows:
Percentage Tyrosinase Inhibition = (A(Control) – A(Test)) / A(Control) x 100%
The variable A(Control) represents the absorbance of the control solution. On the other hand, A(Test) refers to the absorbance of the plant extract and fractions tested at different concentrations. The linear regression analysis determined the inhibitory concentrations at which the absorbance reaches 50% (IC50) for the tyrosinase inhibition.
Acetylcholinesterase inhibition assay
The acetylcholinesterase inhibition assay was performed according to the methodology described by Shahrivari et al. (2022) to assess the inhibitory potential of MCME and its fractions. A volume of 140 µL of a 0.1 M sodium phosphate buffer solution at pH 8.0 and 7.0 was combined with 20 µL of plant extract and fractions at various concentrations ranging from 1000 µg/mL to 15.625 µg/mL. Additionally, 15 µL of a 0.28 U/mL solution of AChE enzyme was added to the mixture. The resulting solution was kept for 15 min at room temperature. Next, 10 µL of a 0.075 M solution of acetylcholine iodide (ATCI) and 10 µL of a 0.01 M of dithionitrobenzoic acid (DTNB) were added under dark conditions. The absorbance measurement was obtained using a microplate reader at a wavelength of 412 nm. The equation for calculating acetylcholinesterase (AChE) inhibition is as follows:
Percentage AChE Inhibition = [(Blank Value - Sample Value) / Blank Value] x 100.
Brine shrimp lethality test
The lethal effects of various concentrations of MCME and its fractions on brine shrimp were assessed according to the method described by Zahra et al. (2021). The plant samples were reconstituted in 1% dimethyl sulfoxide (DMSO). Artemia salina eggs (0.5 g) were sprinkled in synthetic seawater (3.8% w/v sea salt) at pH 7 for 48 hours to hatch the shrimp eggs. Five milliliters (5 mL) of various sample concentrations (62.5 µg/mL - 1000 µg/mL) were added to the wells containing 5 mL sea salt solution. Ten nauplii were transferred in each well and were incubated at room temperature under constant light for 24 hours. After the incubation, LC50 values and percentage mortality of nauplii were calculated. The experiments were repeated three times.
GC-MS analysis
The GC-MS analysis was conducted using an Agilent, 19091S-443, USA instrument equipped with an HP-5MS column (30 m 0.25 mm, with a film thickness of 0.25 µm) to analyze the chemical compositions of the MCME and most potent fractions. The oven temperature was set to 70°C and maintained for 3 min. It was then gradually increased to 120°C and held for 2 min. Subsequently, the temperature was further raised to 150°C and maintained for 2 min. Finally, the temperature was increased to 240°C and held for 5 min. The injector and transfer line temperatures were configured to 230°C and 240°C, respectively. A volume of 1 μL of the diluted samples was injected into the GC-MS instrument. Bioactive compounds were identified by comparing their mass spectra with analogous compounds from the NIST libraries (Al-Dhahli et al., 2020).
Molecular modeling
The crystal protein structure of AChE (PDB ID: 6O52) (Gerlits et al., 2019) was retrieved from the protein data bank (https://www.rcsb.org/) (Kurumbail et al., 1996). The homology model of human tyrosinase was built using the crystal structure of tyrosinase from Priestia megaterium (PDB ID: 6QXD) (Ielo et al., 2019). The protocol used was described by Zengin et al. (2022). The two proteins were prepared using the PlayMolecule webserver. A program that predicts the pKa of the titratable residues and uses it to protonate each protein. The three-dimensional structure of each ligand was obtained from the PubChem database (https://pubchem.ncbi.nlm.nih.gov), and geometry optimization was performed using UCSF Chimera (Pettersen et al., 2004). All prepared proteins and ligands were converted into Protein Data Bank, Partial Charge (Q), and Atom Type (T)) (PDBQT) format using MGLTools 1.5.6 (https://autodock.scripts.edu). Docking was carried out using AutoDock vina (PyRx) (https://vina.scripps.edu/) (Allouche, 2009). with the docking grid coordinates for human AChE (X= -0.0074 Å, Y = 39.14 Å, Z = 2.07 Å) and for human tyrosinase (X = 8.84 Å, Y = 14.28 Å, Z = 121.37 Å). The binding energy scores were calculated for each ligand pose (exhaustiveness = several runs: 8) against each protein and the best pose was analyzed using Biovia DS Visualizer v4.5 (BIOVIA, San Diego, CA, USA).
Table 1
Antioxidant activity of M. cajuputi leaf extract and fractions.
S/no. | Sample | DPPH IC50 (µg/mL) |
1. | MCME | 28.14 ±0.96 |
2. | MF1 | 35.26 ±0.64 |
3. | MF2 | 80.90 ±0.17 |
4. | Ascorbic acid | 7.40 ± 0.47 |
Table 2
Enzymes inhibition potential and toxicity study of M. cajuputi extract and fractions.
Table 3
GC-MS analysis results of MCME
Table 4
GC-MS analysis result of MF1
Peak No. | R.T.(min.) | Compound name | Molecular formula | Total area % |
1. | 8.214 | γ-Eudesmol | C15H26O | 9.21 |
2. | 8.447 | α-Eudesmol | C15H26O | 18.83 |
3. | 10.237 | β-Eudesmol | C15H26O | 71.96 |
Table 5
GC-MS analysis result of MF2
RESULTS AND DISCUSSION
The findings demonstrated that the crude extract of M. cajuputi leaf and its fractions (MF1 and MF2) possess promising antioxidant and enzyme-inhibitory properties. However, MF3 and MF4 showed no activity in all the bioassays. While fractions MF1 and MF2 did not exhibit any toxicity effect, MCME demonstrated mild toxicity on Artemia salina. These results indicated that the bioactive compounds from M. cajuputi leaf could be used as alternative antioxidants and enzyme inhibitors. It has been reported that polyphenols, tannins, terpenes, and alkaloids are plant-active components and exogenous antioxidants with promising efficacy (Pandey et al., 2022).
Antioxidant activity
The antioxidant properties of MCME and its fractions, as determined by DPPH free radical scavenging assays, are presented in Table 1. The MCME exhibited significant antioxidant activity with the IC50 value of 28.14 µg/mL, whereas fractions MF1 and MF2 demonstrated moderate antioxidant activity, with IC50 values of 35.26 µg/mL and 80.90 µg/mL, respectively, and the reference compound (ascorbic acid) had an IC50 value of 7.40 g/mL. Phytochemical analysis of the MCME revealed the presence of terpenes compounds and phenolics, which could be attributed to its remarkable free radical scavenging activity (Paari, Pari, Kannan, & Gunaseelan, 2019). According to a report, the antioxidant activity of natural products is significantly impacted by their phenolic contents (Al-Abd et al., 2015). The results of this study corroborate the antioxidant activity of Melaleuca species by Khalaf et al. (2021), which scored IC50 values ranging from 60.97 μg/mL to 34.60 μg/mL. It has been highlighted that natural products could be regarded as potential alternative antioxidants, as synthetic antioxidants are associated with adverse effects (Jia et al., 2023). Consequently, the leaf of M. cajuputi could be considered a valuable source of natural antioxidants for industrial applications.
Enzyme inhibition and toxicity studies
The enzyme inhibition and toxicity effects of MCME and its fractions are presented in Table 2, and the lethality curves for the BSLT are obtainable in supplementary material, Appendix A . Based on the results, it was observed that MCME demonstrated significant anti-tyrosinase activity (IC50 value of 65.81 µg/mL), followed by MF1 (IC50 value of 131.3 µg/mL) and MF2 (IC50 value of 215.3 µg/mL). The positive control (Kojic acid) demonstrated an IC50 value of 198.4 µg/mL. Tyrosinase is an essential enzyme in melanogenesis. Therefore, inhibiting these enzymes is an important treatment strategy for hyperpigmentation disorders (Shahrivari et al., 2022). One of the most critical concepts in drug development for hyperpigmentation disorders is suppressing the tyrosinase enzyme by natural competitive inhibitors to avoid the skin-related issues associated with synthesized compounds like hydroquinones and kojic acid (Cheraif et al., 2020). The anti-tyrosinase activity of MCME could be due to the terpenes and phenolic compounds identified in the extract. The results are consistent with that of Saber et al. (2021), who reported the anti-tyrosinase activity of Feijoa sellowiana (a member of the Myrtaceae family) with an IC50 value of 115.85 mg/mL. Flavonoids and phenolic substances have been shown to have considerable tyrosinase inhibitory activity (Saidi et al., 2019). Thus, the enormous bioactive components identified in the plant extract and fractions such as 3,4,5-trimethoxybenzoic acid, 2-isopropyl-10-methylphenanthrene, α-eudesmol, and 2-tert-butylanthracene could be responsible for the observed inhibitory effects. Interestingly, MCME and MF1 showed better anti-tyrosinase activity than kojic acid (positive control). Therefore, M. cajuputi leaf might be an alternative source of tyrosinase inhibitors.
The results of the anticholinesterase assay indicate that MF2 demonstrated the highest potency against cholinesterase enzyme, with an IC50 value of 3.35 μg/mL. Followed by MF1 with an IC50 value of 26.7 μg/mL. The least activity was observed on MCME (IC50 value of 282.6 μg/mL). The IC50 value for the control compound (Galantamine) was 5.50 μg/mL. Alzheimer's disease is usually managed by inhibiting AChE activity, which helps restore cholinergic stability (Shahrivari et al., 2022). Compared with galantamine (positive control), MCME and MF1 exhibited weak inhibitory activity on AChE. However, it is noteworthy that MF2 showed higher efficacy than galantamine. Sareedenchai, Petrachaianan, Chaiyasirisuwan, Athikomkulchai, and Sareedenchai (2019) reported a remarkable percentage inhibition of the AChE enzyme by M. cajuputi essential oil (21.18 ± 0.54%). Similarly, oxygenated monoterpenes derived from Mentha pulegium and Lavandula officinalis exhibited remarkable AChE inhibition (Cheraif et al., 2020). The strong anti-cholinesterase activity of MF2 could be attributed to the major compounds 10-methylanthracene-9-carboxaldehyde and 2-isopropyl-10-methyl phenanthrene or the synergistic effect of all the bioactive components. The observed cholinesterase inhibition by MCME and fractions suggests that M. cajuputi has the potential to be a cognitive enhancer and may aid in the management of Alzheimer's disease.
On the other hand, the toxicity testing of the MCME and fractions on Artemia salina showed that MCME had LC50 values of 781 μg/mL, whereas MF1 and MF2 recorded LC50 values of 6621 and 1165 μg/mL, respectively. According to Clarkson’s toxicity classification index, LC50 more than 1000 µg/mL is considered non-toxic, LC50 between 500 µg/mL and 1000 µg/mL is termed low toxicity, whereas from 100 µg/mL to 500 µg/mL is moderately toxic and high toxicity is recorded at less than 100 µg/mL (Noor, Yusuf, Wahab, Adam, & Sul’ain, 2020). In light of these considerations, MCME (781 µg/mL) showed low toxicity, whereas MF1 (6621 µg/mL) and MF2 (1165 µg/mL) were non-toxic against Artemia salina. In a previous study, it has been reported that MCME showed toxicity at LC50 427 µg/mL. However, M. cajuputi essential oil (MCEO), M. cajuputi aqueous extract (MCAE), and M. cajuputi decoction extract (MCDE) with LC50 values 2131.1, 1062, and 2477 µg/mL respectively were non-toxic (Noor et al., 2020). In contrast, Daud et al. (2018) reported that MCME had no toxic effects on the reproductive systems of male and female Sprague Dawley rats, perhaps due to several factors like varying solvent percentage, simultaneous physiological changes of the extract, and different exposure times in experiments.
The GC-MS analysis of MCME, MF1, and MF2
The total ion chromatograms (TIC) of MCME are shown in Figure 1. A total of thirty compounds were identified. Based on the TIC profile, 11 prominent peaks were observed within the retention time range of 5.92 to 12.74 minutes, as indicated in Table 3. Valencene (sesquiterpenes) occurred at a retention time of 8.45 min and recorded the highest total area percentage (19.61%). The GC-MS analysis of MF1 identified three compounds, as presented in Table 4. The total ion chromatogram (TIC) of MF1 is shown in Figure 2. These compounds include β-eudesmol (71.96%), α-eudesmol (18.83%), and γ-eudesmol (9.21%). The GC-MS analysis of MF2 identified five compounds, as indicated in Table 5. The total ion chromatograms (TIC) are shown in Figure 3. The major compound is 2-isopropyl-10-methylphenanthrene, with a total area percentage of (83.09%). The presence of these bioactive compounds in the leaf extract of M. cajuputi corresponds with previous findings that reported similar phytoconstituents in M. cajuputi leaf extracts (Hassan et al., 2022; Sahimi et al., 2022). However, minor differences in the total area percentages of the compounds could be influenced by factors including geographical location, harvest time, plant parts used, and extraction processes (Hassan et al., 2022). These bioactive compounds can act synergistically or independently to provide various pharmacological effects, such as enzyme inhibition, antimicrobial, anti-inflammatory, and antioxidant properties (Cheraif et al., 2020). For example, the antioxidant activity of natural products like plant extracts is significantly linked to their phenolic contents (Al-Abd et al., 2015). Furthermore, flavonoids and phenolic substances such as 3,4,5-trimethoxybenzoic acid have been shown to have considerable tyrosinase and AChE inhibitory activity (Saidi et al., 2019). Terpenes compounds were also highlighted as novel antioxidants and enzyme inhibitors (Cheraif et al., 2020).
Figure 7
Interaction between the human tyrosinase model (built using PDB ID:6QXD) and 2-Isopropyl-10-methylphenanthrene
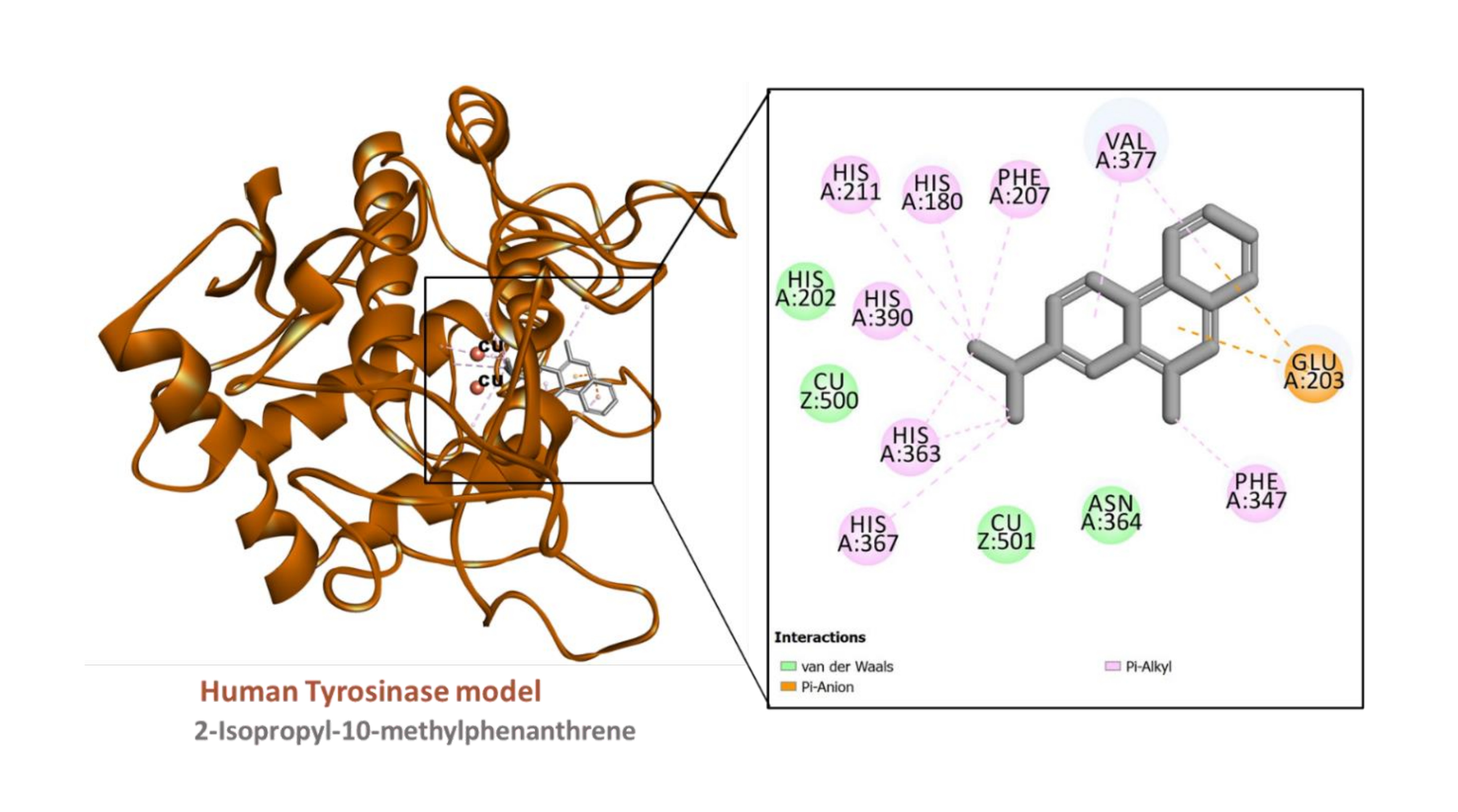
Figure 8
Interaction between the human tyrosinase model (built using PDB ID:6QXD) and 2-tert-Butylanthracene
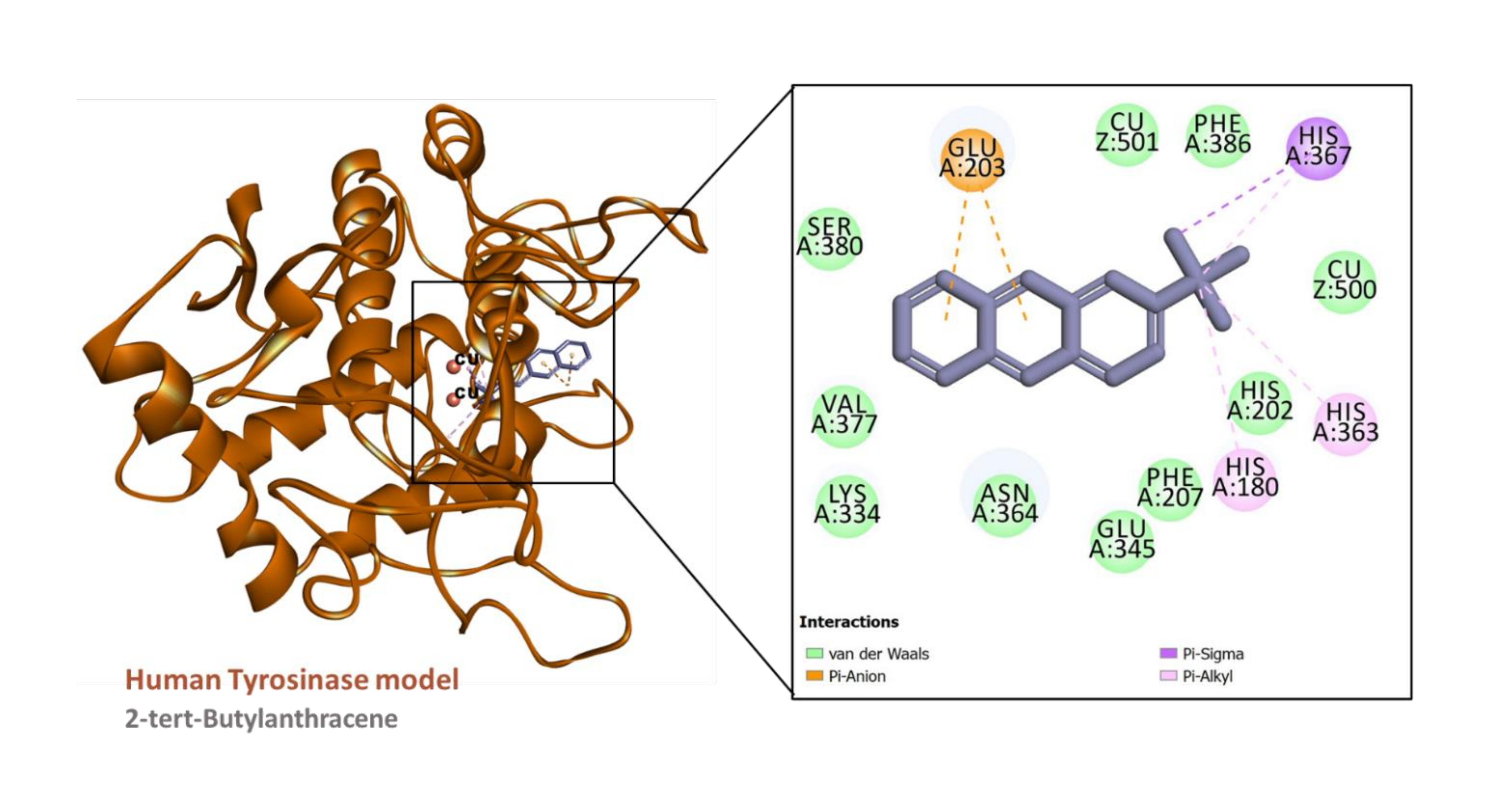
Table 6
Binding energy scores (kcal/mol) of the compounds towards the target enzymes
Molecular docking studies
The predicted binding energy scores suggest that all the ligands studied potentially bound to AChE and tyrosinase (Table 6) and Figure 4. Analysis of ligand-enzyme interaction in some selected complexes reveals the details of the interaction mode. For instance, 10-methylanthracene-9-carboxaldehyde bound to the AChE, mainly via π-sigma interactions with Tyr124 and Tyr341; a π-π stacked interaction with Trp286; hydrophobic interactions with Tyr124, Tyr341, and Trp286; as well as a few van der Waals interaction with amino acids residues lining the active site (Figure 5). The interaction between AChE and β-eudesmol is rather interesting: one H-bond with Tyr72, a π-sigma interaction with Trp286, two hydrophobic interactions with Leu76 and Tyr341, with two van der Waals interactions reinforcing the binding (Figure 6). The key interactions between tyrosinase and 2-isopropyl-10-methylphenanthrene are multiple hydrophobic contacts with residues deep inside the pocket and π-anion interactions with Glu203 near the entrance to the active site. In addition, the two catalytically essential Cu2+ ions, along with a couple of residues, were found to be involved in van der Waals interactions (Figure 7). It is also interesting to note that 2-tert-butylanthracene engaged tyrosinase via two π-anion interactions with Glu203, three hydrophobic contacts with His180, His363, and His367: a π-sigma interaction with His367. Moreover, the two active site Cu2+ ions and several amino acid residues were involved in van der Waals interactions with the molecules (Figure 8). This suggests that the bioactive compounds in M. cajuputi leaves extract and fractions potentially bind to and inhibit the activities of AChE and tyrosinase.
The binding scores from the molecular docking analysis demonstrate a strong correlation with the inhibitory potential of MCME and its fractions. The MCME and MF1 had higher inhibitory activity against the tyrosinase enzyme. Similarly, their active components showed a strong binding affinity for the enzyme, as evidenced by docking scores ranging from -5.1 kcal/mol to -6.2 kcal/mol. In the same vein, the compounds 10-methylanthracene-9-carboxaldehyde and 2-isopropyl-10-methyl phenanthrene, identified in MF2, had a strong affinity for AChE, as indicated by their respective docking scores of -7.6 and -7.4 kcal/mol. This finding provides evidence for the effectiveness of MF2 in inhibiting the activity of AChE. A previous study reported the binding affinities of the standard compounds kojic acid and galantamine towards tyrosinase and AChE, with docking scores of 5.4 kcal/mol and 7.6 kcal/mol, respectively (Dilshad et al., 2022). The findings of this study are similar to previous reports on the inhibitory potential of triterpene, steroid, and flavonoid compounds on tyrosinase and AChE enzymes (Saber et al., 2021; Yırtıcı, Ergene, Atalar, & Adem, 2022).
CONCLUSION
In conclusion, this is the first comprehensive study highlighting the antioxidant, anti-tyrosinase, anti-cholinesterase, and possible mechanism of action of M. cajuputi leaf extract and fractions. The results suggest that MCME and its fractions have significant potential as a source of active compounds with protective properties. Thus, it has promising applications to act as a co-adjuvant in cosmetics and neurodegenerative disease management. Further research is needed to isolate and purify the active components responsible for biological activities.
CONFLICTS OF INTEREST
Given his role as Associate Editor, Gokhan Zengin has not been involved and has no access to information regarding the peer review of this article. Full responsibility for the editorial process for this article was delegated to Editor-in-Chief Carlos L. Cespedes Acuña. There are no relevant conflicts of interest regarding this article.
Author contributions
Musa Isah - Collection and/or assembly of data and writing the article. Gokhan Zengin - Critical revision of the article. Wan-Nor-Amilah Wan Abdul Wahab - Research concept and design. Hasmah Abdullah - Critical revision of the article. Mohd Dasuki Sul’ain - Final approval of the article. Abdullahi Ibrahim Uba - Data analysis and interpretation. Wan Rosli Wan Ishak- Research concept and design. Shajarahtunnur Jamil-Data analysis and interpretation.