Introduction
Chronic diseases represent a significant cause of increased mortality and morbidity cases worldwide. The number of cancer patients is expected to reach 24 million in 2035, as estimated by the International Agency for Research on Cancer (IARC) (Bray, Ren, Masuyer, & Ferlay, 2013). In Sudan, although there is no national, systematic screening programme for cancer registry, the number of diagnosed cancer cases is increasing (Elamin, Ibrahim, Abuidris, Mohamed, & Mohammed, 2015; Elebead, Hamid, Hilmi, & Galal, 2012). Furthermore, the number of malaria patients was estimated by the WHO as 229 million cases worldwide in 2019, with more than 1.8 million cases from Sudan (OCHA, 2019; WHO, 2020) (WHO, 2020; OCHA, 2019). Several oxidative stress-induced pathological disorders and disease conditions, including diabetes, cancers, aging, neurological, and cardiovascular disorders, generate free radicals (Ramana, Reddy, Majeti, & Singhal, 2018). Antioxidants play key roles in counteracting oxidative stress by protecting cells against the effects of free radicles. Natural antioxidants are generally categorized into dietary sources, including vegetables, fruits, cereals, and beans and those from medicinal plants and wild herbs (Wilson et al., 2017). Studies have indicated that antioxidants have a high potential to inhibit the development of many diseases, including cancer (Ramana et al., 2018). Nowadays, the pharmaceutical industries are searching for potential natural and safe raw materials to overcome the side effects of synthetic drugs and problems related to drug-resistant (Abdin et al., 2020). Many investigated plant extracts and isolated phyto-components have a wide range of pharmacological properties and could be used as the safest, most effective and natural source instead of chemical drugs. Karunakaran, Firouz, Santhanam, and Jong (2020) isolated two anticancer compounds (ananixanthone and trapezifolixanthone) from Calophyllum macrocarpum and proved their cytotoxicity against HeLa Chang liver and HEK-293 cell lines. Also, some Omani Hapllophyllum tuberculatum species recorded potent antioxidant and intense cytotoxic activities (Al-Muniri & Hossain, 2017). Furthermore, several examined plant extracts exhibited considerable effect against Plasmodium parasites, as displayed by Syzygium guineense and Parinari congensis extracts (Laryea and Borquaye, 2019) and evaluated by leaf extract of Fadogia cienkowskii (Orabueze, Adesegun, Ejeatuluchukwu, Ota, & Coker, 2019). Therefore, plants are considered a potential natural source for new lead antimalarial and antitumor compounds and antioxidant agents. The diversity of climates in Sudan results in a wide variety of flora species which comprises about 3137 plant species belonging to 170 families and 1280 genera (Khalid, Abdalla, Abdelgadir, Opatz, & Efferth, 2012). Considering all the issues mentioned above and in an attempt to explore plant-based alternative solutions in promoting health, the objective of this study was to examine 14 medicinal plants, traditionally used in Sudan to cure different diseases, for their antioxidant, antimalarial, and cytotoxic potential and to determine their total phenolic and flavonoid contents. These plants are; Aerva javanica (Burm.f.) Juss. ex Schult., Burnatia enneandra Micheli, Cissus quadrangularis L., Coccinia grandis (L.) Voigt, Dioscorea dumetorum (Kunth) Pax, Dioscorea hirtiflora Benth., Fagonia arabica L., Geigeria alata Benth. & Hook.f. ex Oliv. & Hiern, Leptadenia arborea (Forssk.) Schweinf., Maerua pseudopetalosa (Gilg & Gilg-Ben.) DeWolf, Mitragyna inermis (Willd.) Kuntze, Raphionacme splendens Schltr., Striga hermonthica (Delile) Benth., and Tephrosia apollinea (Delile) DC. The identities and traditional medicinal uses of the investigated plants are compiled in Table 1.
Table 1
Profile of the investigated plants
Materials and methods
Plant materials
Samples of the selected plants were collected from different regions in Sudan. The plants were identified and authenticated at the Department of Botany, Faculty of Science, University of Khartoum, by Maha Y. Kordofani. Voucher numbers are given in Table 1.
Preparation of extracts
Samples were dried in the shade at ambient temperature and then grounded into fine powder. Fifty grams of each plant material was macerated in chloroform: methanol (1:1 v/v) solvent for 24 hours, then filtrated and concentrated using a rotary evaporator under reduced pressure and weighed.
Determination of total phenolic (TPC) and (total flavonoids (TFC) contents
The TPC and TFC were determined by adopting the method described by Wolfe, Wu, and Liu (2003) and Ordoñez, Gomez, Vattuone, and Lsla (2006). The detailed procedures were given in the Appendix A.
Pharmacological evaluation
Antioxidant activity of the extracts was estimated using DPPH in vitro method (Wang, Yang, & Zhang, 2007), ABTS radical-scavenging activity (Re et al. (1999)Re et al. (1999) and Ferric reducing antioxidant power (FRAP) (Benzie and Strain (1996)Benzie and Strain (1996). The antiplasmodial activity was determined by the in vitro microplate assay against the NF54 strain of Plasmodium falciparum (sensitive to all known drugs). A modification of the [3H] hypoxanthine incorporation assay was used (Witschel, Rottmann, Kaiser, & Brun, 2012). Cytotoxicity of the extracts, at concentrations of 50 and 100 μg/mL, was performed as described by Mosmann (1983) using the thiazolyl blue tetrazolium bromide (MTT) procedure. The detailed pharmacological procedures were given in the Appendix A.
Statistical Analysis
All experiments were performed in triplicate, and the results were expressed as mean ± standard deviation (SD) values. Significant differences between samples were analyzed using analysis of variance (ANOVA) and Duncan’s multiple range test (P< 0.05).
Table 2
Phenolic contents and antioxidant activity of extracts of the investigated plants.
Results and Discussion
Plant bioactivity and medicinal aspects are usually linked with various classes of secondary phytochemicals such as phenols, flavonoids, terpenes, etc. Their presence and concentration vary from one plant to another and even from part to another in the same plant, mainly used in Ethnobotany (Kumar et al., 2019).
Phenolic content
Phenolics are well recognized to exert several biological properties, including antioxidant, antiproliferation, and anticarcinogenic activities (Wilson et al., 2017). Accordingly, the total polyphenolics and flavonoids contents of the studied plant's extracts were determined (Table 2). The values of total polyphenolic content were ranged between 11.78 ± 0.07 to 94.43 ± 0.63 μg gallic acid eq/mg extract. G. alata exhibited the highest phenolic content, followed by D. hirtiflora (66.45 ± 0.30 μg gallic acid eq./mg), L. arborea (stem) (57.25 ± 1.37 μg gallic acid eq./mg), C. quadrangularis (56.01 ± 0.64 μg gallic acid eq./mg), M. inermis (51.25 ± 0.51 μg gallic acid eq./mg) and S. hermonthica (51.05 ± 0.07 μg gallic acid eq./mg). Other species extracts had total polyphenolic content of less than 40 μg gallic acid eq./mg.
The total flavonoid content values in the extracts ranged between 2.63 ± 0.03 to 31.81 ± 0.74 μg quercetin eq./mg extract. The extract of S. hermonthica showed the highest flavonoid content followed by that of G. alata (30.20 ± 0.79 μg quercetin eq./mg extract), L. arborea (stem) (25.32±1.66 μg quercetin eq./mg extract), L. arborea (leaf) (24.88±0.41 μg quercetin eq./mg extract), C. quadrangularis (24.52±0.65 μg quercetin eq./mg extract), Aerva javanica (22.86±0.95 μg quercetin eq./mg extract) and D. dumetorum (19.92±0.26 μg quercetin eq./mg extract) respectively. Other species extracts showed total flavonoid content < 11 μg quercetin eq./mg extract.
Antioxidant activity
The antioxidant capacity for the selected 14 plant extracts was evaluated using three complementary assays; DPPH, ABTS and FRAP (Table 2). Different extracts exhibited various free radical scavenging activities, as indicated by their IC50 values. In the DPPH assay, the IC50 values of plants extracts ranged from 13.23 to 1233.24 μg/mL. C. grandis displayed the highest DPPH scavenging activity, followed by S. hermonthica (IC50 28.14±0.25 μg/mL), D. hirtiflora (IC50 47.28±3.24 μg/mL), M. inermis (IC50 66.69±1.12 μg/mL), G. alata (IC50 68.54±0.71 μg/mL) respectively. The IC50 values of different plant extracts ranged from 35.54 to 712.72 μg/mL from the ABTS assay. The highest ABTS scavenging activity was obtained from G. alata, followed by D. hirtiflora (IC50 59.9 μg/mL), S. hermonthica (IC50 71.21 μg/mL) and M. inermis (IC50 73.19 μg/mL) respectively. From the FRAP assay values (were ranged from 43.24 ±0.62 to 107.15±0.11 nmol Fe+2 eq./mg where S. hermonthica exhibited the highest FRAP reducing power (107.15 ± 0.11 nmol Fe+2 eq./mg), followed by B. enneandra (106.31 ± 0.92 nmol Fe+2 eq./mg), A. javanica (100.71 ± 0.4992 nmol Fe+2 eq./mg), L. arborea (stem) (99.76 ± 3.42 nmol Fe+2 eq./mg), C. quadrangularis (96.38 ± 2.69 nmol Fe+2 eq./mg), G. alata (83.13 ± 0.86 nmol Fe+2 eq./mg) and M. inermis (82.69 ± 1.18 nmol Fe+2 eq./mg) respectively.
It was clear that extracts displayed variable antioxidant capacity in different assays. Although both ABTS and DPPH assays are associated with electron and radical scavenging, the studied extracts revealed variable capacity in both assays. This observation has been reported previously, where it was suggested that factors like stereo-selectivity of the radicals or the solubility of the extracts in different testing systems affect the capacity of extracts to react and quench other radicals (Jimoh, Adedapo, & Afolayan, 2010). The observed high antioxidant activity of some plant extracts may be attributed to high concentrations of phenolic compounds with potential protective effects (Spanou, Stagos, Aligiannis, & Kouretas, 2010). Furthermore, these findings supported previous results on the antioxidant activity of S. hermonthica (Kiendrebeogo, Dijoux-Franca, Lamien, Meda, & Wouessidjewe, 2005), C. grandis (Deshpande, Patil, Parmar, Daswadkar, & Khodade, 2011), C. quadrangularis (Prabhavathi, Prasad, & Jayaramu, 2016; Tiwari, Gupta, & Sharma, 2021), D. hirtiflora (Adeniran, Sonibare, Ajayi, Rajacharya, & Kumar, 2020; Sonibare & Abegunde, 2012), G. alata (Zheleva-Dimitrova et al., 2017) and M. inermis (Ouédraogo et al., 2020) using different extraction solvents.
Antimalarial activity
In vitro antimalarial activity was measured using the chloroquine-sensitive NF54 strains of Plasmodium falciparum (Table 3). Of the 14 plant species, only B. enneandra showed considerable in vitro antiplasmodial activity with an IC50 value of 5758 ɳg/mL. However, except for determining total phenolic content, this plant has never been investigated for its secondary metabolite content. Other plants extract showed IC50 value >10 000 ɳg/mL. However, some of these plant species are reported to be used to treat malaria in other countries and were found to exert antiplasmodial activity in other plasmodial strains (Philip, Elizabeth, Cheplogoi, & Samuel, 2017; Tefera & Kim, 2019).
Table 3
In vitro inhibition of plant species extracts under the study against Plasmodium falciparum strain NF54.
For example, leaves of M. inermis is used to treat malaria in Guinea, and an antiplasmodial activity test on strain W2 showed that it has potent activity with an IC50 value of 4.32 µg/mL (Traoré et al., 2015). Leaf of C. grandis possessed antiplasmodial activity against P. falciparum with an inhibition value of 69 % (Ravikumar, Inbaneson, & Suganthi, 2012). S. hermonthica exhibited a high intrinsic antimalarial activity (68.5% suppression of parasitaemia) against P. berghei in mice (Okpako & Ajaiyeoba, 2004). Dichloromethane extract of C. quadrangularis whole plant had moderate (IC50 23.9 µg/mL) antiplasmodial activity against P. falciparum chloroquine-sensitive strain 3D7 (Bah, Jäger, Adsersen, Diallo, & Paulsen, 2007). Leaves and roots of D. dumetorum are commonly used to treat malarias in Southwest Negeria (Dike et al., 2012), but no scientific evidence was found. Moreover, pure compounds, tephroglabrin, (-)-semiglabrin, semiglabrinone, (+)-glabratephrin isolated from T. apollinea were found to be inactive against P. falciparum (Khalid, Farouk, Geary, & Jensen, 1986). Thus, it could be concluded that the antimalarial activity of plant extracts depends on many factors, among them the type of extract and plasmodial strains.
Figure 1
Cytotoxicity of 15crude extracts of investigated plants towards HCT-116, MCF-7, MDA-MB-231 andEAhy-296 cells lines at concentrations 50 and 100 μg/mL for 48 hours.
A.j, Aerva javanica; B.e, Burnatia enneandra; C.q, Cissus quadrangulari;C.g, Coccinia grandes; D.d, Dioscorea dumetorum; D.h, Dioscorea hirtiflora; F.a, Fagonia arabica; G.a, Geigeria alata; L.s & L.l, Leptadenia arborea (s:stem, l:leaf);M.p, Maerua pseudopetalosa; M.i, Mitragyna inermis; R.s, Raphionacme splendens; S.h, Striga hermonthica; T.a, Tephrosia apollinea.
Different superscript letters indicate significant difference (p<0.05).
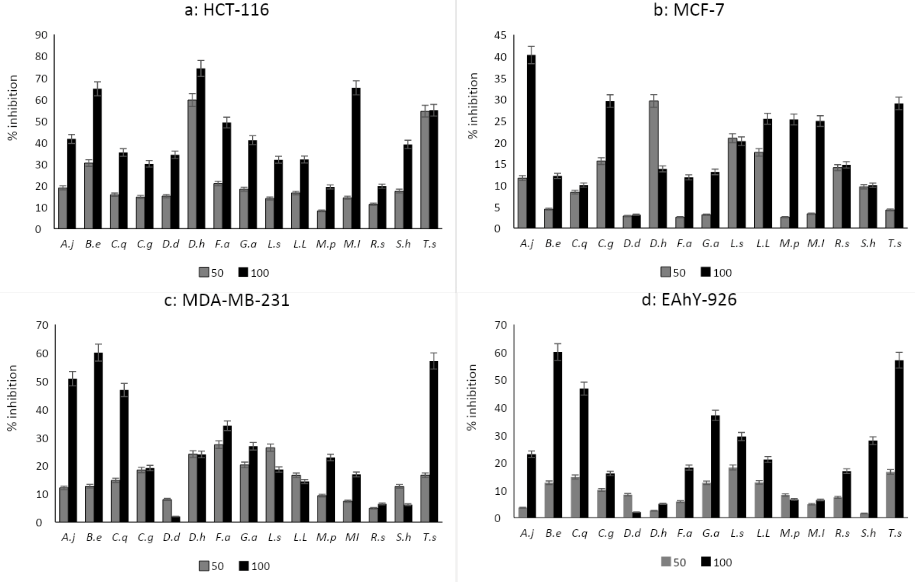
Cytotoxicity
The cytotoxicity of the 15 plant extracts against three cancerous cell lines, HCT 116, MCF 7 and MDA-MB-231, in addition, to one human normal cell line, EAhy 926, were determined after treatment with 50 and 100 μg/mL of extracts for 48 hours (Figure 1). Cytotoxicity of extracts against HCT 116 showed that at 50 μg/mL, D. hirtiflora displayed cytotoxicity with inhibition values of 59.59 ± 13.03%. At 100 μg/mL, the same extract displayed the highest cytotoxicity (74.23 ± 03.72%), followed by those of M. inermis (65.28 ± 04.60%) and B. enneandra (64.79 ± 02.05%) respectively (Figure 1-a). All the 15 studied extracts did not exert cytotoxicity against the MCF-7 cells line at both concentrations used (Figure 1-b). Extract of A. javanica was the only extract with toxicity against the MDA-MB-231 cell line with an inhibition value equal to 50.82 ± 07.46% at 100 μg/mL (Figure 1-c). Furthermore, all extracts were not toxic to human normal cell line EAhy-926 at 50 μg/mL. Nevertheless, at 100 μg/mL, B. enneandra and T. apollinea extracts were relatively toxic (60.04 ± 01.21% and 57.07 ± 03.11%, respectively), while all other extracts were not toxic (Figure 1-d).
The results of cytotoxicity screening of the present study revealed that D. hirtiflora and M. inermis displayed interesting cytotoxic effects towards HCT -116. Both plant species were not toxic to the normal EAhY-926 cell line. No published data on the antitumor activity of D. hirtiflora, A. javanica and B. enneandra were found. However, the antitumor effect of other Dioscorea spp like D. bulbifera (Wang et al., 2012), D. esculenta (Murugan & Mohan, 2012), and D. opposite (Liu et al., 2016; Zhang, Wang, Liu, & Li, 2016) was reported. A previous study on M. inermis showed that the alkaloid rich extract did not exert mutagenic or genotoxic activity (Traore et al., 2000)
Conclusion
In conclusion, the results obtained in this study showed that total polyphenolic and flavonoid contents and antioxidant activity differ among extracts of the studied medicinal plants. Some of these medicinal plants are promising sources of natural antioxidants. Results of antiplasmodial activity demonstrated that B. enneandra could be a potential source of antimalarial agent(s). Cytotoxicity screening suggested that D. hirtiflora and M. inermis are promising candidates with antitumor activity. It is well known that plant extracts comprise mixtures of complex metabolites, which exert their action on different levels and through several mechanisms. Thus, isolation and determination of bioactive molecule(s) responsible for the antitumor activity of these two species and their mode of action are warranted to evaluate their potential for cancer therapy. Moreover, bioassay-guided fractionation of crude extracts of B. enneandra would be interesting to determine and verify whether the same constituents or different ones exert their toxicity towards cancer and normal cells. To the best of our knowledge, this is the first report on the cytotoxicity property of these two plants and the antiplasmodial activity of B. enneandra.
Author contributions
IBEEB, SY, AAAA, AMA - Research concept and design; IBEEB, MYK, AAQ, EMAS - Collection and/or assembly of data; MYK, AAAA, AAQ, EMAS - Data analysis and interpretation, SY, AAQ, AMA, MAAM, AMAA - Writing the article; SY, AMA, MAAM, AMAA, GZ - Critical revision of the article, GZ - Final approval of the article.
Conflicts of interest
Given his role as Associate Editor, Gokhan Zengin has not been involved and has no access to information regarding the peer review of this article. Full responsibility for the editorial process for this article was delegated to Editor-in-Chief Carlos L. Cespedes Acuña. All authors reported that there is no conflict of interest associated to this work.