INTRODUCTION
The liver is the largest and most important vital organ of the human body. It plays a crucial role in the management of many physiological processes associated with several essential processes like metabolism, secretion, and storage. It has a propensity to detoxify endogenous (waste metabolites) and exogenous (toxic chemicals) compounds of the organisms (Mani et al., 2022). In addition, metabolic processes of development, nutrient provision, energy production, reproduction, bile secretion, vitamin storage, and the metabolism of fats and carbohydrates are also carried out by the liver. Because of all, hepatic disorders are still one of the biggest health challenges and these are serious public issues around the globe (Hajdarevic, Vehabovic, Catic, & Masic, 2020).
The liver is negatively affected by biological factors, autoimmune, toxic chemicals [carbon tetrachloride (CCl4), aluminium chloride (AlCl3)], drugs [paracetamol (PCM), thioacetamide, dimethylnitrosamine (DMN), diclofenac, isoniazid, valproic acid, d-galactosamine/lipopolysaccharide (GalN/LPS)], infections, disturbances in the blood supply and unquestionably, excessive consumption of alcohol (Singh, Cho, & Upadhyay, 2016). Excessive exposure to toxins and dosage of pharmaceuticals results in altered homeostatic status that leads to reactive oxygen species (ROS) generation. Altered ROS generation increases lipid peroxidation, oxidative stress and inflammations leading to organ malfunction that resulting hepatic steatosis, hepatitis, cirrhosis, haemochromatosis, cholestatic and hepatocellular carcinoma (Singh et al., 2016).
Drug-induced liver injury (DILI) and herb-induced liver injury (HILI) are well-known concerns that can symptomatically reflect both acute and chronic liver disorders. The clinical manifestations of DILI are similar to HILI. It occurs due to exposure to chemical drugs either produced naturally or synthesized in the laboratory. Annually, out of 10.000-100.000 people about 10-15 people suffered from hepatotoxicity after taking prescripted or OTC medicines (Raschi & Ponti, 2015).
Despite significant advances in modern medicine, there are no entirely effective drugs that improve liver function, offer complete protection for the organ, or facilitate the regeneration of hepatic cells. Although the majority of synthetic drugs for liver diseases are effective pro-oxidant scavengers, their prolonged use could have substantial adverse effects. (Farzaei et al., 2018). Taking this into consideration, researchers are inclined to employ plant-based drugs because they will have greater efficacy, be more cost-effective, and have little to no toxicity. Therefore, there has long-established empirical evidence for using herbal treatments to alleviate hepatic problems; grapefruit and its polyphenols are one of them (Ibrahim et al., 2022). Cited literature reported that grape (fruit/seed/peels) contains a huge number of polyphenols that are capable of significantly modulating the levels of alanine phosphatase and transaminase (ALP and ALT), aspartate transaminase (AST), thiobarbituric acid-reactive substances (TBARS), malondialdehyde (MDA), lipid peroxides (LPO), protein carbonyl content, total bilirubin (TLB), lactate dehydrogenase (LDH), and enzymes increasing the activity of catalase (CAT), glutathione peroxidase (GPx) glutathione (GSH), superoxide dismutase (SOD), alcohol dehydrogenase (ADH), and aldehyde dehydrogenase (ALDH), as well as the levels of vitamins C and E in the hepatocytes (Madrigal-Santillán et al., 2014; Wiegand & Berg, 2013).
In the current scenario, numerous studies have been reported on the hepatoprotective potential of grapes and polyphenols. However, we have presented a comprehensive systematic analysis of grapefruit/seed/peel and polyphenolic compounds based on rigorous inclusion and exclusion criteria for their hepatoprotective potential.
METHODS
Search Strategy
According to PRISMA guidelines, a systematic review was done to find the information available for grapes and polyphenols for hepatoprotective activity. The following keywords were used: “Hepatotoxicity” OR “Hepatoprotective” OR “Liver injury” AND “Hepatotoxic drug” AND “Grape” OR “Grapefruit” OR “Grape seed” OR “Grape peels” OR “Bioactive compounds” OR “Polyphenols” AND “Preclinical studies” OR “Clinical studies”.
Study Selection
PubMed, Google Scholar, Springer, Scopus, Taylor & Francis, and Science Direct were searched for studies published online without language and time restrictions. We have limited our search to focus on the hepatoprotective potential of grapefruit/seeds/peels and bioactive compounds especially polyphenols, whereby only online research papers having complete studies are considered. Based on rigorous inclusion and exclusion criteria total of 65 studies were included in the current study and Mendeley was used as a database manager (Figure 1).
RESULTS
Figure 1 summarizes the research selection procedure as per PRISMA principles. Using the online database search, a total of 184 records were retrieved. After 79 duplicate publications were excluded, 105 papers were included, and further 27 papers were rejected based on the full text, title and/or abstract. Moreover, 13 papers were removed for not meeting the inclusion criteria of the eligible studies (n = 78) read. Finally, 65 papers were included after the selection procedure was complete.
Rationale for the use of grape and polyphenols for the management of hepatotoxicity
For thousands of years, plants have been used as sources of medicine, grape is one of them. Grapefruit/seeds/peels contain a variety of polyphenols that could serve as the foundation for the development of future medicine. These may be used for hepatoprotection either alone or in combination with synthetic medicine, making them an attractive target for the future (Nada et al., 2015; Saha et al., 2019). By putting forth this effort, existing liver disease medicines may be given a wider range of potential applications and it makes sense that liver disease treatments will be cured using grapes in their entirety or their bioactive components. Because of their natural origin, cultural acceptability, minimal or no side effects, affordability, and accessibility, plant-derived medicines have been utilized for the management of various diseases for a long time, with reportedly inconclusive findings for improving outcomes in developed and developing countries. Many medicines derived from plant origin to manage various diseases and disorders including atropine, aspirin, artemisinin, resveratrol, ephedrine, digoxin, reserpine, quinine, cannabidiol, vinblastine, vincristine, dronabinol, and many more (Amedei & Niccolai, 2014). Hence, to replace currently prescribed drugs with dubious efficacy and safety, we need to look for novel, inexpensive, and effective plant-based therapeutics for the treatment of liver illnesses.
Mechanisms of conventional drug-induced hepatotoxicity
The liver lesion is indicated by hepatocytes damage, which manifests by elevation in ALT, AST and cholestatic lesion when ALP increases twice than actual. Hepatocytes, cholangiocytes, ductal cells, Kupffer cells and endothelial cells are all involved in the processes by which drugs cause hepatotoxicity; they also directly affect cellular organelles such as the endoplasmic reticulum, mitochondria, cytoskeleton, microtubules, or nucleus (Guicciardi, Malhi, Mott, & Gores, 2013; Morales et al., 2016; Xu et al., 2020). Moreover, the development of drug metabolites in the liver through biotransformation may result in hepatic injury due to toxic chemicals or free radicles compounds and may trigger several chemical reactions (Xu et al., 2020). These pathways may cause apoptosis, necrosis, or both. The primary culprits of liver damage include the following mechanism mentioned below and examples of some conventional drug-induced hepatotoxicity (Table 1).
Table 1
Some conventional drugs induce hepatotoxicity
Drug | Possible Mechanism of hepatotoxicity | References |
Acetaminophen | Through the CYP450 system, it is converted to hazardous intermediates. High acetaminophen doses lower GSH levels or stop the pathway; this intermediate accumulates and binds to intracellular macromolecules that can cause cell damage, typically by caspase-independent apoptosis started by PARP-1 activation. | |
Aspirin | Direct intrinsic hepatotoxic. Inhibit mitochondrial function, elevates serum ALT levels and mild bilirubin | |
Dabigatran | Hepatic injury due to marked elevation in enzymes in the liver | Rochwerg, Xenodemetropoulos, Crowther, and Spyropoulos (2012) |
Glipizide | Liver damage may be exacerbated by hypersensitivity. Hepatocellular, cholestatic, and mixed injuries have all been linked to sulfonylurea-induced liver damage. | |
Interferon beta (ß-1a and ß-1b) | Chronic hepatitis is suggested by persistent ALT increases. | |
Isoniazid, rifampicin, pyrazinamide | Lipid peroxidation (necrosis). Formation of hepatotoxic metabolites | |
Ketoconazole | Potentially inhibited human CYP 3A4, which can alter the serum levels of many drugs that are metabolized via the P450 system and ultimately increase liver toxicity. | |
Lovastatin | Lovastatin is largely metabolized by CYP 3A4 and metabolites are excreted in bile. The mild ALT elevations are likely due to a toxic metabolite. The onset of clinical injury (usually cholestatic, but can be hepatocellular) can vary from weeks to years. | |
Methimazole | The liver injury occurs due to an increased level of serum ALT | Gomez-Peralta, Velasco-Martínez, Abreu, Cepeda, and Fernández-Puente (2018) |
Methotrexate | Direct toxicity. Lipid peroxidation (necrosis). Dysfunction of mitochondrial and increase in liver enzymes | |
Methylphenidate | It is extensively metabolized in the liver and has many drug-drug interactions as well as increases serum aminotransferase level | |
Olanzapine | Olanzapine has extensive hepatic metabolism, partially by CYP450 enzymes and some cases of clinically apparent hepatotoxicity may be due to the production of a toxic metabolite and increased level of serum ALT | |
Orlistat | Hypersensitivity is likely to be the cause of liver injury | |
Phenytoin | DILI is caused by phenytoin due to a hypersensitivity reaction, mostly in typical cases of immunoallergic hepatotoxicity. It is metabolized by the CYP450 system to arene oxide, which may result in toxic or immunogenic metabolite formation. | |
Rifampin (Rifampicin) | The cause of injury is likely to be due to toxic metabolic products that directly induce an immunologic reaction. | |
Tacrolimus | Liver injury related to serum enzyme elevation. | |
Tamoxifen | Disrupt β-oxidation of lipids. Steatohepatitis, necrosis, and inflammatory infiltrate due to higher levels of ALT | |
Valproic acid | It lowers tissue carnitine levels, which can lead to inhibition of beta-oxidation and loss of mitochondrial function, hyperammonemia and microvesicular steatosis. | |
Vancomycin | Direct toxicity or immune adverse reactions |
Mitochondrial dysfunction: It may occur due to the disruption of β-oxidation of lipids and oxidative energy production within the hepatic cells. In addition, steatosis may exist as a result of aberrant mitochondrial activity and fat accumulation, which can also result in hepatic apoptosis, ATP depletion and consequent necrosis, and mitochondrial membrane permeabilization or rupture.
Immune response: The creation of new antigens is believed to be responsible for the immune response, resulting cause idiosyncratic hepatotoxicity. Additionally, it may be characterized by inflammatory cells like neutrophils and lymphocytes.
Oxidative stress (OS): The liver is the most frequent target organ for drug toxicity. It has been suggested that the production of free radical species, specifically ROS and RNS, is an early sign of drug toxicity and a sign of its propensity to be harmful to the liver. Additionally, OS is generated when ATP is depleted and the concentration of calcium inside hepatic cells increases, which may result in liver necrosis.
Lipid peroxidation: Free radicals and fatty acids in the membranes interact to cause lipid peroxidation, which might result in the formation of electrophilic metabolites that can damage cellular DNA.
Clinical manifestations
In both acute and chronic use, several medications cause liver damage. DILI can relate to either asymptomatic liver injury or liver failure which is clinically severe. However, the most reliable sign of a drug's propensity for severe hepatotoxicity is an increase in blood TBL and aminotransferase levels. The liver enzymes that are most frequently upregulated by the use of modern medicines without any clinical signs are AST followed by ALT and ALP (Figure 2) (Bessone et al., 2021). The identification of a higher rate of these elevations in drug-using participants compared to a placebo group is a sensitive signal of the potential to generate severe DILI. According to the published reports, a higher rate of the more pronounced peak in ALT elevations (10x, 15x upper limit of normal-ULN), with cases of rises to >1,000 U/L raises more worries and is considered a definite indicator of hepatotoxicity (Devarbhavi, 2012).
Figure 2
Diagrammatic presentationof hepatotoxicity in response to conventional drugs and protective effect ofgrape and polyphenols. Kupffer and endothelial cells are activated by harmfulchemicals and chemotactic molecules secreted by injured hepatocytes. Theseoccurrences could lead to hepatocyte stress, which is exacerbated by thepresence of ROS and NO species produced by active endothelial cells.Hepatocyte death and Kupffer cell fibrosis enhancement are the end results.Nf-kB: IL-1: Interleukin-1; IL-6: Interleukin-6; I-1β: Interleukin 1beta; IFNγ:Interferon-gamma; JNK: nuclear factor erythroid 2–related factor 2: Nrf2; NO:nitrogen oxides; TGF-β: Transforming growth factor beta.
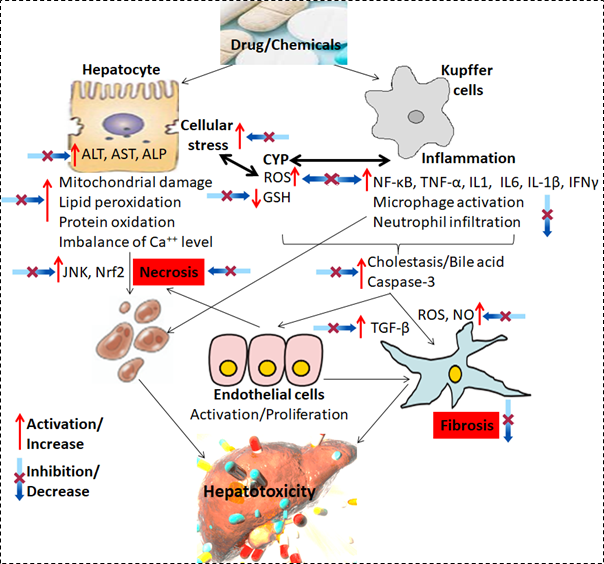
Another potentially helpful indicator of liver function that might point to DILI is an elevated plasma prothrombin (PT) time or its international normalised ratio as a result of decreased hepatic synthesis of vitamin K-dependent clotting components. However, the occurrence of clinically severe liver injury or impaired liver function (with prolonged PT or hypoalbuminemia) that leads to deficient protein synthesis is rare (Figure 2). Jaundice, gastrointestinal discomfort, itching, and other symptoms are among them (Fernandez, Lenoir, Samer, & Rollason, 2021). In addition to medications, DILI also covers injuries caused by dietary supplements, herbal remedies, and medicinal plants.
Therapeutic potential of grape and polyphenols
Diets are essential for improving health care and preventing disease and disorders because they contain a huge number of bioactive molecules. Grapes are well known for their useful remedial functions as they are rich in polyphenols and have beneficial health effects. Some important polyphenols found in grapes like quercetin, gallic acid, catechin, syringic acid, naringenin and many more play a key role to protect liver disorder, oxidative stress, inflammation, brain disorders, diabesity, cardiovascular disease and disorders etc., (Li et al., 2018; Nada et al., 2015; Saha et al., 2019).
The hepatoprotective mechanism of grapes and polyphenolic compounds is assumed to be an elevation in antioxidant enzyme activities, down-regulation of CYP2E1, iNOS, CASP-3, and NF-kB expression, up-regulation of trace element concentration levels, and activation of SIRT1 gene expression, which results in an improvement of the antioxidant and anti-inflammatory status in the hepatic tissues (Ismail, Salem, & Eassawy, 2016). A previously published report suggested that ethanolic extract of grape impressively inhibited the level of serum ALP and TLB, whereas resveratrol significantly reduced oxidative stress, hepatic inflammation (IL-1, IL-6, NF-kβ, TNF-α, and IKK), and apoptosis markers CASP-3, CASP-9, and Bax] in streptozotocin-induced diabetic rats (Giribabu, Karim, Kilari, Kassim, & Salleh, 2018). Moreover, grape polyphenols reduce severe inflammation either by regulating inflammatory pathways or by reducing ROS levels. Grape flavonoid derivatives may a better remedial choice than synthetic ones because they are natural constituents that can choose numerous paths to reduce chronic inflammation (Haghighatdoost, Gholami, & Hariri, 2020). According to another study, grape seed extract controls LDH leakage from liver cells while maintaining antioxidant enzyme levels. The enrichment of bioactive substances such as polyphenols, vitamin E, and ascorbic acid may be responsible for these actions (Dogan & Celik, 2012). Although several polyphenols are cited in the literature, few of them are described. Caffeic acid exhibited potential anti-inflammatory and antioxidant qualities and is successful in the treatment of severe liver damage. By engaging with the Nrf2 binding site, inhibiting it from binding to the liver cancer factor kelch-like ECH-associated protein-1 (Keap1), and increasing the expression of key antioxidative signals like HO-1, it can alter Keap1's expression (Yang et al., 2017). Quercetin scavenges ROS, and inhibits xanthine oxidase as well as lipid peroxidation, in addition to scavenging and stabilizing iron. It also reduces the level of ALT and AST which will help to minimise liver damage (Barros et al., 2017).
Table 2
Grape and polyphenolic compounds used for the management of hepatotoxicity against hepatotoxic drugs and chemicals
Constituents | Dose | Mechanism | Inducing agents | References |
---|---|---|---|---|
Grapefruit/seed/peel | 27ml/kg/b.w./rat | Attenuates the level of ALT and AST and protects against liver damage. | AlCl3 | |
200mg/kg/b.w./rat | Ameliorates the activity of MDA, GSH, and SOD activity in liver cells and protects against cell injury | LPS/ d-GalN | ||
200-600 mg/kg/b.w./rat | Reduced the activities of ALP, ALT and AST | Paracetamol | ||
Conventional/organic juice | Modulates CAT, SOD and GPx activities. Also, inhibit hepatocellular degeneration and steatosis | High fat diet | ||
100 mg/kg/b.w./mice | Diminished elevated levels of ALT, AST, and MDA while enhancing GSH, SOD and CAT levels. Protect infiltration, irregular nuclei and altered mitochondria in live tissue | Ehrlich solid tumor | ||
100-200 mg/kg/b.w./rat | Significantly reduced MDA, NO contents in liver homogenates and AST, ALT, ALP, and TLB levels in serum. While significantly increased serum GSH content in liver homogenate. Attenuates disruption of the hepatic architecture and collagen fibres deposition in the portal tract | Thioacetamide | ||
100 mg/kg/b.w./rat | Significantly decrease MDA level and efficiently increases GSH level and catalase CAT, SOD activity. Attenuated liver injury to normal | Diethylnitrosamine | ||
150 mg/kg/b.w./rat | Decreased AST, ALT and LDH serum enzyme levels and MDA contents in liver tissues | Ethanol | ||
Gallic acid | 50 mg/kg/b.w./rat | It increases antioxidant enzyme (SPD, CAT) activities, decreased plasma hepatic enzyme (ALT, ALP, AST) and pro-inflammatory markers (IL-1β, IL-6, COX-2, TNF-α) | CCl4 | |
100 mg/kg/b.w./mice | Modulates AST, ALT, ALP, lipid peroxidation and TNF-α levels in serum and antioxidant status (OSD, CAT and GSH) | PCM | ||
20 mg/kg/b.w./rat | Diminished hepatic damage via upregulated hepatic mRNA expression of GLUT-4, Wnt1 and β-catenin with inhibitory effects on the elevated expression of ERK1/2/NF-κB | STZ | ||
100 mg/kg/b.w./rat | Attenuating the inflammatory mediators COX-2 through the NF-κB inhibition pathway increases SOD and ATPases (Ca2+/Mg2+) expression | N′-Nitrosodiethylamine | ||
10-20 mg/kg/b.w./rat | Decreased level of TBARS and MDA in liver homogenates. Improve antioxidant status and liver injury. | NaF | ||
Catechin | 50-100 mg/kg/b.w./rat | Prevented mitochondrial membrane damage, up-regulation of p53, Bax and down-regulation of Bcl-2 mRNA levels in the liver. Protect hepatic cell damage. | d-GalN | |
100-400 mg/kg/b.w./mice | Increased SOD, CAT, GX, and GSH levels and markedly decreased the MDA level. Protect liver cell damage | CCl4 | ||
Epicatechin | 50-100 mg/kg/b.w./mice | Decrease the level of ALT, AST, LPO, IL1β, TNF-α, and NO while increasing CAT, SOD and GSH levels. Reduced histological damage. | MTX | Azadnasab, Kalantar, Khorsandi, Kalantari, and Khodayar (2021) |
20 mg/kg/b.w./rat | Declined MDA level whereas GSH, CAT, GST, GPx, and CYP450 levels were upregulated. Regenerated damage of hepatic tissue. | CCl4 | ||
Quercetin | 50-100 mg/kg/b.w./rat | Reduction of ALT, AST and amelioration of liver damages. | PCM | |
10 mg/kg/b.w./rat | Protect the liver against DNA damage in mild liver injury | ACR | ||
70 mg/kg/b.w./rat | Enhance the level of SOD, and GPx while decreasing AST, ALT, LPO, direct bilirubin, and TLB level and also protect tissue damage | ACR | ||
20 mg/kg/b.w./rat | Decreased level of MDA and increased GSH and CAT activities. Protect liver injury | CCl4 | ||
50 mg/kg/b.w./rat | Increases level of SOD, CAT, GPx, and GSH while decreasing MDA level. | PCM | ||
50mg/kg/b.w./mice | Attenuated the NF-κB, TNF-α, COX-2, TGF-β1 and expression of iNOS | CCl4 | ||
Kaempfrol | 20mg/kg/b.w./mice | Decreased AST, ALT, LPO, TG, and MDA levels in serum and increases the level of SOD, and GSH. Reduced the expression level and activity of hepatic CYP2E1 | Alcohol | |
50mg/kg/b.w./mice | Reduced ALT, AST activities, and increased GSH, SOD, GPx, CAT and ameliorates liver injury | CCl4 | ||
250 mg/kg/b.w./rat | Increased GSH, Bcl-2 levels while decreasing the level of MDA, ROS, TNF-α, Bax. It also reduced the expression of CYP2E1, and the activity and protein levels of SIRT1. | APAP | ||
Rutin | 20 mg/kg/b.w./rat | Reduced ALT, MDA and total bilirubin while increasing GSH, SOD, GPx and CAT levels. Attenuates liver cell damage through uniform mitochondrial distribution, regeneration of rough ER, and vacuolations in the cytoplasm | APAP | |
40 mg/kg/b.w./rat | Decreased level of MDA, ALP, AST and ALT while increasing SOD and CAT. Attenuates infiltration of hepatic tissue | Isoniazid | ||
10-250 mg/kg/b.w./rat | Attenuated the NF-κB, TNF-α, COX-2, TGF-β1 and expression of iNOS | CCl4 | ||
70 mg/kg/b.w./rat | Downregulates expression levels of IL-6, Bcl-XL, MEK5, FADD, EGF, STAT3 and JAK while upregulates the Bcl2 expression | CCl4 | ||
50 mg/kg/b.w./rat | Decreased level of AST, ALT, LDH, ALP and CASP-3 while increasing GPx and GSH | 5-FU | ||
40 mg/kg/b.w./rat | Decreased ALT activity, TLB, TNF-α), LPO, NFκB and Increased hepatic GSH level. | d-GalN | ||
Chlorogenic acid | 60 mg/kg/b.w./rat | Inhibited the activities of mitochondrial NADH dehydrogenase and ATP synthase and the contents of ATP, and AMP in the liver and protect the liver | LPS | |
50mg/kg/b.w./mice | Decreased the elevated plasma levels of ALT and AST and also suppressed hepatic mRNA expression of toll-like receptor 4 (TLR4), TNF-α and NF-kβ p65 subunit | LPS | ||
60-200 mg/kg/b.w./mice | Decrease the serum levels of AST, ALT, TC, TG, and total albumin and elevated the serum total albumin and the activities of GSH, CAT, SOD, GSH-Rd, and GSH-Px increased. Decreased the formation of lesions in liver | CCl4 | ||
| Protect liver injury markers (AST, ALT and ALP), hepatic inflammatory cytokines (IL-1, IL-6, TNF-α and TNF-β) and dyslipidemia (TC, TG and LDL-C) | High l-carnitine | ||
100 mg/kg/b.w./mice | Significantly reverse in LPO, enzymatic leakage, Cyt P450 inactivation and produced enhancement of cellular antioxidant defence |
| ||
Resveratrol | 200 mg/kg/b.w./mice | Increased SOD protein and mRNA expression levels in hepatic cells | Ethanol | |
20 mg/kg/b.w./rat | Ameliorates the level of AST and ALT, MDA while increasing the activities of SOD, CAT, GPx and protects against serious congestion and haemorrhage related to the hepatic tissues injury | Cadmium | ||
Syringic acid | 10mg/kg/b.w./mice | Attenuates the level of AST, and ALT and protects liver fibrogenesis | CCl4 | |
50-100 mg/kg/b.w./rat | Effectively reduced AST, ALT, ALP, LDH, MDA, and ROS while increasing SOD and GSH levels. Attenuated inflammatory injury by suppressing TNF-α, IL-1β and NF-κB and also down-regulates CASP-3 expression in the liver | Thioacetamide | ||
Caftric acid | 40 mg/kg/b.w./rat | Decreased the level of AST, ALT, ALP, and MDA while increasing SOD, Cat and GPx | Methamphetamine | |
Caffeic acid | 5 mg/kg/b.w./rat | Increases endogenous level of GSH, GPx, SOD, and CAT | Mercuric chloride | |
3-12 mg/kg/b.w./rat | Markedly decreased MDA levels while increased GSH, CAT and SOD activities in liver | CCl4 | ||
Ellagic acid | 50-100 mg/kg/b.w./mice | Attenuates centrizonal necrosis, fatty changes and liver cytochrome P450 enzymes. Also improve the level of LPO, GSH and SOD | PCM | |
40 mg/kg/b.w./rat | Enhance the level of SOD and CAT in liver tissue. Ameliorates degree of liver fibrosis | Diclofenac | ||
60 mg/kg/b.w./rat | A marked decrease in the level of AST, ALT, ALP, MDA, NO and expression of TNF-α and NF-kB. In addition, it significantly increased protect liver damage | Valproic acid | ||
50-100 mg/kg/b.w./mice | Alleviated hepatic antioxidant activities (GSH, CAT, MDA and SOD), pro-inflammatory cytokines levels (IL-6, IL-1β, and TNF-α), genes expressions (Tlr4, Myd88, Cd14, Cox2, Nos2, and Nfκb1). Also, protect the histological architecture of the liver injury | Alcohol | ||
100 mg/kg/b.w./rat | Attenuates liver function (AST, ALT and ALP), antioxidant enzymes (GSH, CAT and SOD and fibrosis-related genes (MMP9 and MMP2) | thioacetamide | ||
Naringenin | 500 mg/kg/b.w./mice | Attenuates hepatocytes architecture by maintaining extended centrilobular necrosis, steatosis and fibrosis. | CCl4 | |
20-80 mg/kg/b.w./rat | Increase the antioxidant status (SOD, CAT, GPx) while decreasing ALT, AST and LOP levels. | Nickel | ||
30-120 mg/kg/b.w./mice | Markedly decreased cytochrome P4502E1 expression, suppressed oxidative stress, inflammation, apoptosis, ALT, AST, and MAPK levels while increasing SOD, CAT and GSH level | CCl4 | ||
100 mg/kgb.w./mice | Mitigated inflammation by suppressing NF-κB and NLRP3 pathways, CASP-3 and p53. Alleviated oxidative stress by inducing Nrf2 and increasing hepatic SOD activity and GSH | LPS/ d-GalN | ||
20-50 mg/kg/b.w./rat | Inhibit hepatic fibrosis by reducing excess collagen accumulation in the liver. Also, inhibited the elevation of serum ALT, AST, ALP, and bilirubin levels | Dimethylnitrosamine |
Catechin possesses anti-hyperlipidemic properties that can be used to treat the clinical symptoms of non-alcoholic fatty liver disorders, where abnormalities in protein and lipid metabolism play a significant role in the pathophysiology of the liver (Pezeshki, Safi, Feizi, Askari, & Karami, 2016). Epicatechin is crucial for the metabolism of lipids in hypercholesteremia and fatty liver disease. It can suppress the activity of crucial liver enzymes including SGPT and SGOT, which exacerbates the abnormalities in the liver (Shanmugam et al., 2017). Ferulic acid has strong antioxidant properties that can stop the free radical activity. By preventing extracellular matrix-related gene expression, it has a dominant anti-cholestatic effect on liver cholestasis. By improving lipid metabolism, it can frequently activate the MAPK or AMPK signalling pathway. According to numerous reports, ferulic acid regulates the expression of key physiological variables, including PPAR- and CPT-1, which is crucial for treating fatty liver illnesses (Saha et al., 2019). Naringenin, a natural flavonoid, possesses antioxidant, anticancer and anti-inflammatory activity. A naturally occurring flavonoid known as naringenin has antioxidant, anticancer, and anti-inflammatory properties. Although naringenin has very low direct antioxidant activity as a scavenger, it aids in the upregulation of Nrf2 pathways and maintains the normal redox of the cell even in clinical situations where prooxidants and reactive oxygen species are generated as a damage mechanism in hepatocytes (Esmaeili & Alilou, 2014; Saha et al., 2019). Resveratol produced an improvement in cellular antioxidant defence and markedly reversed LPO, enzymatic leakage, and Cyt P450 inactivation. increased levels of SOD mRNA and protein expression in hepatic cells increases the activities of SOD, CAT, and GPx while improving the level of AST and ALT, MDA, and protects against significant congestion and haemorrhage associated with the injury to the hepatic tissues. Additionally, resveratrol is linked to a significant decrease in the number of liver enzymes, cytokines, and transcriptional factors like NF-B. Following the activation of its inhibitor IB, it reduces NF-B expression to prevent liver injury (Kapil et al., 1995; Zhang et al., 2015).
Considering the aforementioned fact, it is apparent that gape contains a huge number of hepatoprotective constituents that may protect the liver as a result of their antioxidant, antiapoptotic, and anti-inflammatory activities described in Table 2 and their hepatoprotective mechanism was shown in (Figure 2).
Clinical studies of grape
A double-blind randomized clinical trial revealed that grape consumption increases the total antioxidant capacity in the body which will help to fight oxidative stress and inflammation (Sarkhosh-Khorasani, Sangsefidi, & Hosseinzadeh, 2021). In another study, it is found that grape extract significantly attenuates the body weight and mass index, waist circumference, as well as waist-to-hip ratio and also lower TNF-α, and high sensitivity C-reactive protein levels (Parandoosh et al., 2020). Grape juice decreased LDL cholesterol, oxLDL and apoB concentrations and raised HDL cholesterol and apoA-1 concentrations in healthy as well as hemodialysis-receiving adults (Castilla et al., 2006). In human male smokers, a polyphenol extract from grape seeds decreased the sensitivity of LDL to oxidation (Vigna et al., 2003). Furthermore, a reported study suggested that the intake of grapes or foods rich in polyphenols boosts vascular health, thereby significantly reducing the risk of cardiovascular diseases as well as diabetes mellitus (Rasines-Perea & Teissedre, 2017).
Table 3
Drug-drug (grape) interactions
Drug-drug (grape interaction)
For millennia, medical care has undergone a global transformation for the development of synthetic drugs throughout the globe. Nevertheless, large portions of the populace in poor nations still rely on herbal medicine and its active constituents for their primary care because they thought that herbal medicine is safe and free from any type of side effects. Although therapies involving herbal products are established most of them have not been studied well, and their usage is either not monitored at all or is monitored very poorly. As a result, there is insufficient understanding of their mechanism of action, potential side effects, contraindications, and interactions with currently available conventional medications and functional foods to recommend the safe and sensible use of these medicines (Ekor, 2014).
Grapefruits, including the seeds and peels, are rare in that it is not used therapeutically, but because of the numerous bioactive compounds in grapefruit, it inhibits CYP3A4 might result in drug interactions. Dihydropyridine calcium channel blockers and HMG-CoA reductase inhibitors are the medications most frequently involved. Of the several hundred chemical entities in the grape that are responsible for these interactions. Hence, the safety of any herbal medicine is important as like efficacy and it is needed to assure that all herbal drugs are safe and of suitable quality. Some examples of drug-drug interaction in the context of grapes are presented in Table 3 (Aronson, 2006).
CONCLUSION AND FUTURE PROSPECTIVE
Grapes, one of the most widely consumed fruits in the world and are used in the traditional system of medicine to treat or prevent a variety of diseases. The polyphenols along with vitamins and fibre found in grape skin and seeds can be used as a functional component to treating various health ailments. In the current study, through numerous research studies, it has been established that grapes and polyphenols are the potentials to protect against liver toxicity and they may replace or enhance currently prescribed synthetic drugs for the treatment of hepatotoxicity by adding them to other efficient pharmaceutical formulations.
Herbal medicine including grape or polyphenols, continue to gain recognition and application on a global scale. Contrary to the erroneous belief that herbal medicine is "safe" because they are produced from "natural" sources, issues linked to adverse responses have also recently become more apparent, more common, and unassailable. In order to safeguard the health of the public, it is necessary to ensure the safety and quality of all herbal medications. In the meanwhile, using herbal medicines correctly can have positive therapeutic effects on users with lower or minimal associated risk. However, it should be understood that there are some adverse effects associated with using herbal products. Additionally, the use of adulterated herbal materials and improper formulation must be prohibited because they might result in the production of inferior and life-threatening herbal medications. Though, thorough scientific investigations (both preclinical and clinical), analysis, and suitable laws and regulations should be followed strictly to ensure the efficacy, safety and quality of herbal medicines.
Conclusively, it is plausible to conclude that grapes and polyphenols have a promising future in the healthcare system and may eventually replace or be used as an adjuvant to synthetic medicine for the management of hepatotoxicity.