Introduction
Mushrooms have been recognized as valuable sources of food and medicine throughout history. However, only recently, they have garnered significant attention due to their abundant biologically active substances and nutritional properties. Currently they are evaluated for their nutritional value and pharmacological properties (Wasser, 2010).
Oudemansiella Speg. (Physalacriaceae, Agaricomycetes, Basidiomycota) has been circumscribed to species with dry to generally viscid pileus, that usually include scattered floccules, white to off-white subdistant lamellae with a central stipe, a lack of veil remnants, and a rudimentary or fugacious veil (Alberti, Pérez-Chávez, Niveiro, & Albertó, 2021). The genus is widely distributed throughout tropical and temperate regions, and its basidiomata grow on rotting wood (Petersen, Hughes, & Cramer, 2010). The number of species within Oudemansiella have been reported to range from 25 (Dulay, 2023) to 33 (indexfungorum.org). Seven species have been documented for South America, with Oudemansiella canarii (Jungh.) Höhn. and Oudemansiella cubensis (Berk. and M.A. Curtis) R.H. Petersen as the most conspicuous and common species in the neotropics (Albertí, Niveiro, Zied, & Albertó, 2020; Veloso, Campi, Maubet, & Mancuello, 2021).
Higher Basidiomycete mushrooms contain bioactive compounds in fruiting bodies and mycelia. Several studies already showcased that many of these mushrooms have medicinal properties and activities such as antimicrobial, antiviral, antioxidant, anticancer, immune system enhancer, radical scavenger, anti-parasitic and anti-inflammatory (Wasser, 2017; Zeb & Lee, 2021). However, only a handful of species have been subjected to bioprospecting endeavours aimed at characterizing their chemical and biological profiles or facilitating domestication for intensive cultivation. Notably, Oudemansiella species produce oudemansins and strobilurin, which are compounds recognized for their antifungal activity and that thwart competition from other fungi (Anke, 1997; Niego et al., 2021). Additional notable metabolites include oudenone, utilized to treat hypertension (Hamao, Osamn, & Takenchi, 1974; Tsantrizos & Zhou, 1995), mucidin, an antifungal antibiotic (Nerud, Sedmera, Zouchová, Musílek, & Vondráček, 1982; Šubík, Behúň, & Musílek, 1974), bioactive molecules such as lectin (Liu, Ng, & Wang, 2013; Matsumoto, Natsume, Ueda, Saitoh, & Ogawa, 2001), and polysaccharides (Wang, Jia, Ren, Li, & Zhang, 2018). In terms of their potential use in biomedical applications, Oudemansiella species exhibit potent antimicrobial activity against pathogenic yeasts such as Candida albicans, C. glabrata, C. krusei, C. tropicalis and Cladosporium sphaerospermum (Rosa et al., 2003). Moreover, several studies have reported moderate anticancer and antioxidant properties (Acharya, Nandi, & Dutta, 2019; Rosa et al., 2003; Veloso et al., 2021).
There is a growing interest about the nutritional and pharmacological properties of wild mushrooms for functional food and alternative drug development worldwide (Dulay, 2023). Some Oudemansiella species collected from the wild are known to be edible and a valuable part of regional diets (Magingo, Oriyo, Kivaisi, & Danell, 2004). For example, nutritional characterizations have shown that O. canarii and O. cubensis are excellent sources of nutrients, rich in carbohydrates, proteins, fiber, vitamins, and minerals (Alberti et al., 2021; Dulay, 2023). However, there is scarce information available on the nutritional values of other similar species. Furthermore, there are limited reports regarding safe consumption and their effects on human health.
Anticipating the growing importance of the genus Oudemansiella in biotechnological applications, the objective of this work was to evaluate the biological, chemical, and nutritional properties of the neotropical species Oudemansiella cubensis. In particular, we focused on evaluating its antioxidant, toxicological, mutagenic, genotoxic, and antigenotoxic properties for its potential role in natural therapeutic or pharmaceutical uses.
Materials and Methods
Collection, cultivation, and sample preparation
The wild sample (Figure 1 a) was collected in the Central Department, San Lorenzo city growing on decaying wood and an herbarium reference specimen is kept at FACEN Herbarium, Laboratorio de Recursos Vegetales, Área Micología, Universidad Nacional de Asuncion N°3775 (MC 124). Subsequently, the strain was isolated from the wild fruiting body and codified as FC23 (Figure 1 c). Mycelia were produced from the strain FC23 following (Campi et al., 2023) (Figure 1 d). Additionally, this strain was later used to cultivate fruiting bodies using a sawdust and corn cob substrate perVeloso et al. (2021) (Figure 1 b).
Figure 1
a.Wild fruiting body of Oudemansiella cubensis b. Cultivated fruiting body on sawdust/corn substrate c. Iso latedstrain FC23 d. Mycelium in liquid medium
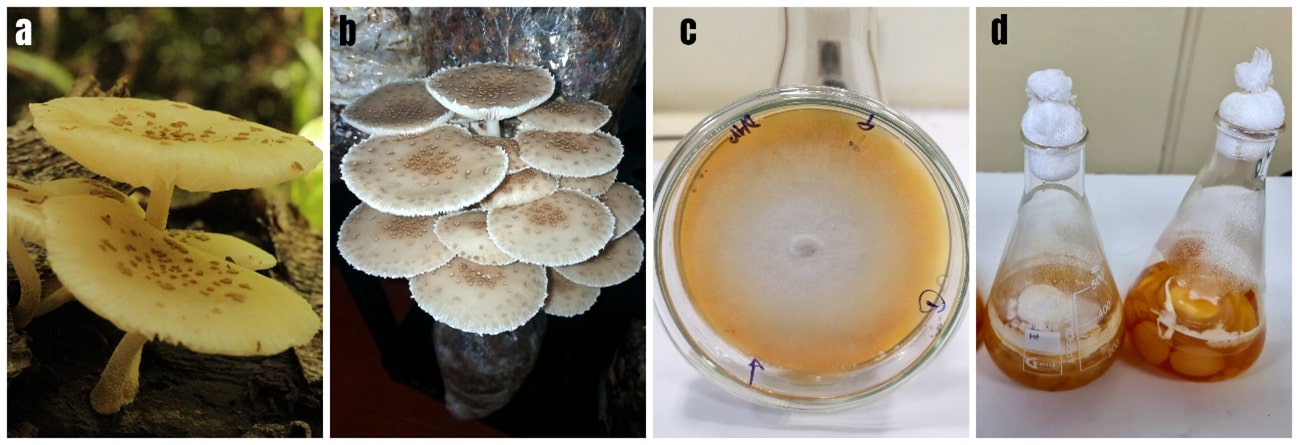
Wild and cultivated fruiting body samples and the mycelium samples were lyophilized (AE-LGJ-12 Freezer Dryer). Fungal material was macerated for two hours with a ultrasonic bath (Digital ultrasonic cleaner PS-50A), then filtered with Whatman filter paper, and the extracts were prepared according to (Campi et al., 2023) while modifying the solvents as ethanol (96%) and ethyl acetate. The extracts were separated from the extraction solvents at 50°C under reduced pressure (RC Ingennery-RE 200A), and any residual water was removed by lyophilization. The resulting samples are later referred to as the ethanolic extract and ethyl acetate extracts, respectively. Genotoxicity and antigenotoxicity studies were performed with ethanolic extracts; antioxidant, total phenolic compounds, antioxidant activity, gas Chromatography-Mass Spectrometry (GC-MS) chemical profile were performed with ethyl acetate extract and antimicrobial activity assay was performed with both extracts.
Total phenolic compounds
Total phenolic compounds (TPC) were determined by UV-VIS spectrophotometry (760 nm) using Folin-Ciocalteu reagent and gallic acid per (Campi et al., 2023). The TPC concentration was quantified as mg.g-1 gallic acid equivalents (GAE).
Antioxidant activity and concentration by the radical scavenging activity assay.
Antioxidant concentrations and activity were determined perCampi et al. (2021) using UV-VIS spectrophotometry (517 nm) and an ascorbic acid standard. The concentration was calculated as:
A = (λDPPH – λSol/λDPPH) × 100
where λDPPH and λSol are the absorbance measurements of DPPH solution and DPPH with extract, respectively.
Gas Chromatography-Mass Spectrometry (GC-MS) chemical profile
Samples for GC-MS were prepared by dissolving wild, cultivated, and mycelium extracts in acetone at an approximate concentration of 1 mg.mL-1. The GC-MS analysis was performed using the Shimadzu GC2010 Plus Gas Chromatograph equipped with a QP2010SE Electron Impact Mass Detector and Supelco SLB-5ms capillary column (I.D. 30 m x 0.25 mm, 0.25 μm). High purity helium (5.0 grade) was the carrier gas operating at a flow rate of 0.87 mL/min and an injection volume of 1.0 μL. The machine settings were set to a 270°C injector temperature, an injection mode of “splitless”, a ratio of 10:1, and a 250°C ion-source temperature. The oven temperature was set to a 2-min, 60°C isothermal with an increase of 6°C/min to 280°C and ending with a 20-min isothermal. The mass detector was programmed in full-scan mode 55-550 m/z at 70 eV. A triplicate analysis was performed. Compounds were identified from NIST database library of the GC-MS instrument. All compounds identified with homology above 90% were considered positive and reported in the results.
Antimicrobial activity assay
Antimicrobial activity was determined by Broth Micro-Dilution Assay following (Campi et al., 2023) and using certificate bacterial and fungal strains Escherichia coli WDCM 00012, Salmonella enterica WDCM 00031, Pseudomonas aeruginosa WDCM 00026, Klebsiella pneumoniae ATCCBAA 1705, Enterococcus faecalis WDCM 00087, Staphylococcus aureus ATCC 6538, S. epidermidis WDCM 00036, and Candida albicans WDCM 00054. The positive control was Meropenem (50 mg.mL-1) for gram negative, Ciprofloxacin (25 mg.mL-1) for gram positive, and Nistatin (20 mg.mL-1) for yeast.
Nutritional composition
The proximal values of the dry matter content was determined from cultivated fruiting body samples. Crude fat content, moisture, ash, protein, and dietary fiber were determined according to guidelines from the Association of Official Analytical Chemists (AOAC, 2000). Crude fat content was determined using hexane for solvent extraction. The crude protein content was calculated using a 4.38 conversion factor per Chang and Miles (2004). The Anthrone method was applied to determine total carbohydrate content with modifications per Campi et al. (2023), which uses glucose anhydrous as the standard. The energetic value was calculated following Mercosur Technical Regulations (Mercosur/Gmc/Res, 2003).
Toxicity testing using median lethal dose (LD50)
Five concentrations (10, 40, 70, 90, and 120 mg.mL-1) of the ethanolic wild fruiting body extract were evaluated for toxicity testing. Solutions of 5 mL for each concentration were prepared and utilized to rehydrate 1.5 g of dried sterile mashed potatoes. These samples were administered to 100 third instar Drosophila melanogaster Canton S[+] larvae for 72 hours. The median lethal dose (LD50) was estimated with Probit analysis based on mortality percentage and the concentration curve transformed to a logarithmic scale (Finney, 1952).
Genotoxicity and antigenotoxicity study determined by the Somatic Mutation and Recombination Test (SMART)
Trans-heterozygous (mwh+/+flr3) Drosophilla melanogaster larvae were produced by crossing 400 virgin females of the flare mutation (flr3 /In(3LR) TM3, ripp sep I(3)89Aa bx34e and BdS) with 200 males of the multiple wing hairs (mwh/mwh) mutation (30). Ethanolic extract samples of wild fruiting body at concentrations of 30, 20, 10 mg.mL-1 were evaluated for genotoxicity by treating 100 third instar trans-heterozygous larvae (mwh+/+flr3) for 72 hours until eclosion, using distilled water as control and 2.61 mg.mL-1 cyclophosphamide as mutagen (Graf et al., 1984).
Antigenotoxicity was assessed for the highest concentration of extract with the lowest amount of mutations obtained in a previous genotoxicity evaluation (20 mg.mL-1). Four different treatments were employed: (i) simple treatment, which involved an oral exposure of third instar trans-heterozygous larvae to extract (20 mg.mL-1), (ii) combined treatment, where third instar trans-heterozygous larvae were orally exposed to extract (20 mg.mL-1) and cyclophosphamide (2.61 mg.mL-1) simultaneously for 72 hours, (iii) chronic post-treatment with extract, where second instar trans-heterozygous larvae were orally administered cyclophosphamide (2.61 mg.mL-1) for one hour followed by extract (20 mg.mL-1) for 72 hours, and (iv) chronic pre-treatment with the ethanolic extract, which consisted of orally exposing first instar trans-heterozygous larvae to extract (20 mg.mL-1) for 72 hours followed by a 48 hours treatment with cyclophosphamide (2.61 mg.mL-1) (Graf et al., 1984) (Figure 2).
Once the treatments were complete, we observed the wings of treated adults with a microscope and identified the number (simple single spot, large single spot, twin spots) and type (mwh or flr3) of mutant cells. The percent inhibition of mutagen activity was calculated using the following equation:
% I = ((TM mutagen – (TM extract + TM mutagen)) / (TM mutagen)) x 100
where TM = total number of mutations.
Statistical analyses
Analysis of Variance (ANOVA) with a 95% confidence interval and Tukey's test were used to compare the variability and statistical significance between the different fractions of the phenolic and antioxidant compounds, antioxidant and antimicrobial activities analyses. The results were expressed as the mean of triplicate measurements ± Standard Deviation (SD). These analyses were performed using the statistical program Past version 4.03b (Hammer, Harper, & Ryan, 2001).
The statistical analysis for the genotoxicity and antigenotoxicity tests were performed based on the frequencies of spots found per wing were compared with control according to the statistical table proposed by Frei and Würgler (1988), which corresponds to a conditional binomial statistical model (Kastenbaum-Bowman test) with significance levels α=β=0.05 (Kastenbaum & Bowman, 1970).
Results and Discussion
Phenolic compounds, antioxidant concentration, and antioxidant activity
The difference of phenolic compound concentrations of O. cubensis FC23 showed Stadistical significance when comparing the ethyl acetate extract from different sources (wild and cultivated fruiting body and mycelia). The cultivated fruiting body extract (36.47 ± 0.51 mg.g–1 GAE) had the highest concentration, followed by the mycelium extract (19.41 ± 0 mg.g–1 GAE) and wild fruiting body extract (10.98 ± 0.32 mg.g–1 GAE) (Table 1). The phenolic compound value for wild fruiting bodies found in our work are consistent to those found in literature for O. cubensis from ethanolic extracts (9.98 mg.g-1 GAE) (Veloso et al., 2021). However, the cultivated fruiting bodies was three times higher than the previously obtained value of 10.38 mg.g-1 GAE (Veloso et al., 2021). Additionally, comparing the methanolic extracts for the mycelia and cultivated fruiting bodies, our values were lower than the 2.38 and 3.88 mg g-1 GAE values reported in literature (Pérez-Chávez, Alberti, Jaramillo, & Albertó, 2017).
Table 1
Phenolic compounds, antioxidant concentration, and antioxidant activity values from extracts of Oudemansiella cubensis FC23.
Previous studies have shown that other species related to O. cubensis, such as O. canarii and O. platensis, resulted in approximate values in the range of 5 mg.g-1 GAE for methanolic extract of wild fruiting bodies (Acharya et al., 2019; Pérez-Chávez et al., 2017), which is twice as low as those obtained for O. cubensis in this work. For the mycelia extract, other work has reported phenolic compound values of 12.86 mg.g-1 GAE for O. canarii aqueous extracts (Contato et al., 2020), which is also lower than observed in this work.
The mean antioxidant compound results ranged between 7.85 to 18.30 mg.g-1 AAE with mean antioxidant activity values ranging from 3.22% to 9.14% (Table 1). The cultivated fruiting body extracts had the highest values, again followed by mycelia and wild fruiting body extracts, respectively, which was consistent with the trend exhibited by phenolic compound values.
Other studies have reported antioxidant compound values of 15.4 mg.g-1 AAE for the ethanolic extracts of wild fruiting bodies of O. cubensis, which is almost twice as much as our results; however, the antioxidant activity (3%) was similar (Veloso et al., 2021). For cultivated fruiting bodies, our antioxidant concentration results were similar to those previously reported for the antioxidants compounds (16 mg.g-1 AAE) but for antioxidant activity, our results were over three times higher than the value (3%) reported in literature (Veloso et al., 2021). These results suggest that the antioxidant activity could be correlated to phenolic compounds concentration.
For related species, such as Oudemansiella platyphyla and Xerula radicata, studies have documented antioxidant activity values of 17% and 5%, respectively (Macáková, 2011). Compared to prior literature, our results suggest that wild and cultivated fruiting bodies and mycelium of Oudemansiella cubensis have slightly higher antioxidant capacity and phenolic content compared to other Oudemansiella species. Nevertheless, the differences in bioactive compound concentrations could be influenced by several factors, such as the extraction procedure, the choice of solvents, the substrate for growing mushrooms, and the liquid culture of the mycelium (Campi et al., 2023).
Table 2
Identified compounds in the wild and cultivated fruiting body and mycelium from the ethyl acetate fraction of O. cubensis FC23.
GC-MS chemical profile
A summary of GC-MS results is shown in Table 2, which includes a list of the identified compounds with their name, molecular weight, retention time, and abundance. GC-MS chromatograms are shown in the Supplementary Materials (Figure S1, S2 and S3 inAppendix A). Various compounds were identified in the ethyl acetate fraction of all wild, cultivated, and mycelia samples. Ethyl acetate fractions presented the best biological activity. Our analysis revealed the presence of fatty acids (saturated, monounsaturated, and polyunsaturated), fatty acid esters, sterols, waxes, phenolic compounds, and vitamins.
For the wild fruiting body, 14 compounds were identified (Table 2). One notable compound with biological activity is l-(+)-ascorbic acid 2,6-dihexadecanoate, which is a fat-soluble vitamin C. The analogues of ascorbic acid formed by esterification and transesterification with fatty acids enhance the antioxidant, anti-inflammatory and antitumor properties of ascorbic acid (Begum, Priya, Sundararajan, & Hemalatha, 2017; Mohamed et al., 2010). Another identified compound worth mentioning is octacosanol, a common natural aliphatic alcohol (Taylor, Rapport, & Lockwood, 2003) that exhibits various biological effects, including anti-fatigue, anti-hypoxia, antioxidant, anti-inflammatory, antitumor, and antibacterial activities against Escherichia coli, Pseudomonas aeruginosa and Bacillus subtilis (Sengupta, Nandi, Bhattacharyya, & Ghosh, 2018; Zhou, Cao, Luo, & Lin, 2022).
In the cultivated fruiting body, the GC-MS assay identified 6 compounds (Table 2). One of these compounds is pyrrolo [1,2-a] pyrazine-1,4-dione, hexahydro-3-(2-methylpropyl), also known as cyclo(l-leucyl-l-prolyl), which is noteworthy for its biological properties. This compound was isolated from Fusarium sp. and showed inhibitory activity against gram-negative and gram-positive bacteria (Putra & Karim, 2020). The antioxidant capacity of cyclo(l-leucyl-l-prolyl) isolated from mushroom Sarcodon aspratus (Berk.) S. Ito has also been reported (Kim et al., 2005). Another compound identified is 7,10-octadecadienoic acid methyl ester, which has been evaluated for cumulative dose-dependent relaxation responses to precontracted penile corpus smooth muscle (PCCSM). This compound was isolated from Calvatia nipponica Kawam., which is known as a natural aphrodisiac (Lee et al., 2020). Additionally, L-(+)-ascorbic acid 2,6-dihexadecanoate was also reported in this fraction.
Finally, 14 compounds were identified in the mycelia extract (Table 2). The most abundant compounds identified were fatty acids and their derivatives, some of these compounds have been reported with antimicrobial activities in nature (Zheng et al., 2005). Linoleic acid (9,12-Octadecadienoic acid, palmitic acid (n-hexadecanoic acid), palmitic acid ethyl ester (hexadecanoic acid, ethyl ester) and ethyl tridecanoate were identified. The linoleic acid (9,12-Octadecadienoic acid), also known as omega-6, has been reported to inhibit some gram-positive bacteria (Dilika, Bremner, & Meyer, 2000).
Table 3
Percentage of inhibition of ethanolic and ethyl acetate extracts from different samples of Oudemansiella cubensis.
Antimicrobial activity
Studies have previously shown that Oudemansiella species exhibit antimicrobial activity (Anke, Besl, Mocek, & Steglich, 1983; Kuhnt et al., 1990; Rosa, Cota, Machado, Rosa, & Zani, 2005). Our results were consistent with results from literature and showed that the ethyl acetate extract generally resulted in better inhibitory activity than the ethanolic extract for the tested microorganisms in Figure 3. This could be due to two possible factors. First, the ability of ethanol to extract polar and non-polar compounds resulting in a complex mixture of metabolites that can exert an antagonistic effect on each other and thus suppress efficient antimicrobial activity (Schmid, Sattler, Grabley, & Thiericke, 1999). Secondly, there is a higher concentration of bioactive metabolites in medium polarity solvents, such as ethyl acetate. Regarding the nature of the wild, cultivated, and mycelia extracts, we have not found a correlation between extract source and antimicrobial capacity. We will further discuss the extracts that showed inhibitory activity (above 50%).
Figure 3
Comparison of antimicrobial activity of ethanol and ethyl acetate extract in wild, cultivated and mycelia samples of Oudemansiella cubensis with notable inhibition of microorganisms. * p < 0.05, ** p < 0.01, *** p <0.001 compared to negative control
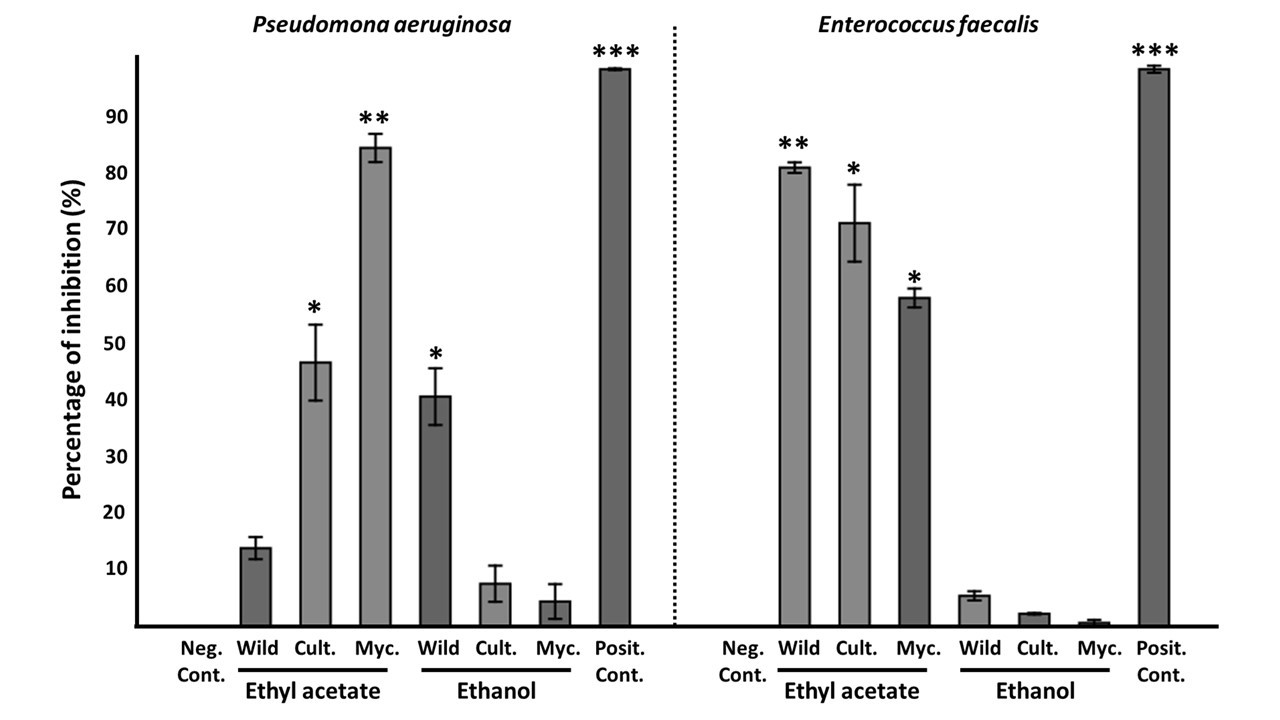
The results from microdilution assay are shown in Table 3. Oudemansiella cubensis exhibited inhibitory activity against Pseudomona aeruginosa 84% (mycelia) and Enterococcus faecalis 71% (cultivated fruiting body) and 58% (mycelia). However, in studies for similar species, Oudemansiella canarii did not show activity against the same microorganisms for the mycelia ethyl acetate extract (Rosa et al., 2003). Additionally, low antimicrobial activity for ethanolic extracts of the fruiting body of O. cubensis against Staphylococcus aureus was found in previous studies (Costa, Santos, & Carvalho, 2021), while our results showed no activity. Previous work also reported inhibition against four Candida species, C. albicans, C. glabrata, C. krusei and C. tropicalis (Rosa et al., 2003), while our study resulted in low activity against C. albicans.
Our results report the presence of compounds with known antimicrobial properties such as l-(+)-ascorbic acid 2,6-dihexadecanoate, cyclo(l-leucyl-l-prolyl), octocosanol and linolenic acid which could explain the inhibitory activity observed in the ethyl acetate fractions of the wild and cultivated fruiting bodies and mycelium. These results support that Oudemansiella is a genus with important antimicrobial properties. Literature additionally mentions isolated molecules of the Oudemansiella genus with antimicrobial activity such as Oudemansin A and B, Dihydroxerulin, Xerulinic acid and Strobilurin C (Anke et al., 1983; Kuhnt et al., 1990; Rosa et al., 2005).
Table 4
Bibliographic comparison of compositions on a dry basis (% dry weight) and energy (kcal/100g) of dried cultivated fruiting bodies of edible Oudemansiella species from literature and this study.
Table 5
SMART test data obtained with the ethanolic extract of O. cubensis evaluated.
Nutritional composition
The approximate nutritional composition of O. cubensis is summarized in Table 4 and was consistent with existing literature of Oudemansiella despite the different substrate used for cultivation. Our analyses revealed that O. cubensis is high in protein (25%) and high in dietary fiber (24%). The fat content for Oudemansiella species is reported to be higher (7-10%) compared to other commercial edible mushrooms (1.7-3.0%) such as Agaricus bisporus, Pleurotus ostreatus, and Agrocybe cylindracea (Alberti et al., 2021). The resulting fat content in this study is also higher (13%) than those reported for O. cubensis from Argentina (10%). A more detailed study of a fatty acid profile would be necessary for a better characterization of the fat content. The protein content was around twice as high compared to O. cubensis and O. canarii from Argentina (Alberti et al., 2021) and O. submucida from Thailand (Table 4). Finally, dietary fiber content found in this work (38%) is similar than those reported in the literature for Oudemansiella species (Table 4). Edible mushrooms are considered as a novel source of dietary fiber, in fact, the functional characteristics of mushrooms are mainly due to the presence of dietary fiber, specifically chitin and beta-glucans (Cheung, 2013; Waktola & Temesgen, 2018).
For carbohydrates, our results obtained with the anthrone method were 12% dry weight. Typically, carbohydrates are a major component in mushrooms with total content ranges from 35% to 70% dry weight (Cheung, 2010; Dimopoulou, Kolonas, Mourtakos, Androutsos, & Gortzi, 2022). However, previous studies of Oudemansiella species reported generally lower values of 23% to 27% (Alberti et al., 2021; Xu et al., 2016; Zhou et al., 2015). Although these results are lower than others, they are incomparable, as those percentages reported in the literature were calculated with the difference method (Alberti et al., 2021; Xu et al., 2016; Zhou et al., 2015). This difference in methods could be the cause for inaccurate determinations of carbohydrate composition in mushrooms (Campi et al., 2023). Additionally, ash content resulted in a lower value (8.6%) than other edible Oudemansiella species. For example, the ash values of O. cubensis obtained are within the values tolerated by some food codes for edible mushrooms (i.e. less than 10% in the Argentine Food Code).
Median lethal dose (LD50), genotoxicity, and antimutagenicity
The toxicity test based on Probit analysis resulted in an LD50 concentration of 37.1 mg.mL-1 (χ2 > 0.05, R2=0.79, P=0.049) of O. cubensis (Figure 4).
Table 5 summarizes the results obtained from experimental treatments for genotoxic and antigenotoxic studies of O. cubensis compared to control groups. The wild fruiting body ethanolic extract showed no genotoxic effect at tested doses of 10 mg.mL-1 and 20 mg.mL-1. Two mutant cell clones were found in samples treated with a an extract concentration of 30 mg.mL-1 (Figure 5). Statistical analysis showed inconclusive results for the assessment of genotoxicity, so the analysis of antimutagenic activity was carried out with the highest concentration that did not shown mutations (20 mg.mL-1).
The total number of mutant cell clones in wings of Drosophila melanogaster flies was variable depending on the given treatment. For the (i) simple treatment, we identified only one mutant cell clone, which agrees with the other characterizations performed in this study. In the (ii) combined treatment, six mutant cell clones were registered with an inhibition of 90.32% of the mutant agent activity. The (iii) chronic post-treatment with ethanolic extract showed no mutant cell clone and 100% of mutagenic activity inhibition. Finally, the (iv) chronic pre-treatment with ethanolic extract revealed five mutant cell clones in the wings of treated flies and showed 91.93% of the mutagenic activity inhibition. These results suggest that ethanolic extract of O. cubensis at the evaluated concentrations (10, 20, and 30 mg.mL-1) has no genotoxicity effect. Additionally, this study reveals an important antimutagenic activity of the ethanolic extract of O. cubensis at the concentration of 20 mg.mL-1 promoting the prevention of the genotoxic damage caused by the mutagenic agent before, during and after mutations were acquired or fixed (Gaivão, Ferreira, & Sierra, 2021; Graf et al., 1984; Graf, Abraham, Guzmán-Rincón, & Würgler, 1998). This damage prevention activity might be related to the presence of antioxidant compounds since there is an argument for a correlation between antioxidant and antigenotoxic activity (Flora, 1998; Kelloff et al., 1994; Malik, Kaur, Prasad, Jha, & Kumar, 2022).
Conclusions
The chemical profile of O. cubensis obtained by GC-MS showcased the presence of compounds such as l-(+)-ascorbic acid 2,6-dihexadecanoate and cyclo(l-leucyl-l-prolyl). Compounds analogous of ascorbic acid contain antioxidant and antitumor properties, which may be attributed to the results of this study. The presence of cyclo(l-leucyl-l-prolyl) also may contribute to the antioxidant properties, as described by other studies (Begum et al., 2017; Kim et al., 2005; Mohamed et al., 2010).
Several studies argue that antioxidant activities are required for natural compounds to demonstrate antigenotoxic/antimutagenic activities, as a mechanism of steps to be performed to obtain chemoprevention by studied molecules against genotoxic damage (Flora, 1998; Kelloff et al., 1994; Malik et al., 2022). Antioxidant activities have also been described in other related species such as O. radicata, O. canarii and O. melanotricha (Dulay, 2023; Pauli, 2010; Wang et al., 2018). This study, despite to be a first approach, has shown that O. cubensis also has antioxidant properties. Deep further studies are needed to, understand and characterize the antioxidant potential and antimutagenic mechanisms.
Oudemansiella is a genus of economic importance and it is already widely utilized as a source of food, cultivated, and marketed in several countries around the world. Oudemansiella cubensis is classified as edible, and our nutritional composition analysis revealed that is rich in protein (25%) and dietary fiber content (24%) while being low in fat (13%). Ethyl acetate and ethanolic extracts of O. cubensis were found to exhibit moderate antioxidant activity and antimicrobial activity against Pseudomonas aeruginosa and Enterococcus faecalis. Additionally, bioactive compounds such as cyclo(l-leucyl-l-prolyl) and l-(+)-ascorbic acid 2,6-dihexadecanoate were identified in the extracts of wild and cultivated fruiting bodies and the mycelium. Finally, O. cubensis also showed important antimutagenic activity in its ethanolic extract.
Funding
This work was financed by the project CONACYT (Consejo Nacional de Ciencias y Tecnología) PINV01-230 “Nuevos recursos endógenos del Paraguay: Bioprospección de compuestos antimicrobianos en hongos lignícolas nativos del neotrópico” and the project PINV01-218 “Caracterización del potencial nutricional y perfil químico de los hongos nativos silvestres, comestibles y medicinales del Paraguay y desarrollo de productos derivados para la industria alimenticia”
Author contributions
CM, MC, EC, GR - Research concept and design, CM, MC, AT, LM, EG - Collection and/or assembly of data, CM, MC, BV, LM, EG - Data analysis and interpretation, MC, YM, EC - Writing the article, MC, YM, EC, BV, GR - Critical revision of the article, CM, MC, YM, EC, BV, GR, LM - Final approval of the article.